Mini-Review on Functional Characteristics of Viili and Manufacturing Process
- 15 November 2023
- Grondin Eric
- (0)
- Probiotics, Scientific paper, Viili
This blog article was written by the authors below and is shared in accordance with the Creative Commons Attribution 3.0 License. No modifications have been made to its English version. If you choose the French language or others, an automatic translation by Google Translate is provided within the article.
Citation : Bakry AM, Campelo PH (2018) Mini-Review on Functional Characteristics of Viili and Manufacturing Process. J Food Biotechnol Res. Vol.2 No.1:7
Copyright : © 2018 by the authors. This article is an open access article distributed under the terms and conditions of the Creative Commons Attribution 3.0 License (https://creativecommons.org/licenses/by/3.0/).
Abstract
Viili (viilia) is ropy and sticky traditional fermented milk that is produced in Scandinavia. In particular, it is popular in Finland. Viili is marked by mesophilic lactic acid bacteria (LAB), fungi and yeasts. The LAB identified in viili including, the acid-producing strains Lactococcus lactis subsp. cremoris and L. lactis subsp. lactis, together with the aroma-producing strains Lactobacillus lactis subsp. Lactis biovar. diacetylactis and Leuconostoc mesenteroides subsp. cremoris. In addition, most traditional viili cultures also contain Geotrichum candidum fungi and several yeast strains including Kluyveromyces marxianus. These microorganisms are being used to produce exopolysaccharides which gives a ropey, gelatinous consistency and a pleasantly mild taste to viili. In addition to its ability to provide a thick body, viili has many of the functional activities including antioxidant, immunomodulatory, ACE Inhibitory and antitumor activity that make it useful in the treatment of cancer, inflammation, diabetes, genotoxicity, accelerated ageing, hypertension and heart diseases.
Keywords: Viili; Fermented dairy; Exopolysaccharide
Introduction
Viili (viilia) is ropey and sticky traditional fermented milk that originated in Scandinavia. In particular, it is popular in Finland [1]. The word viili is from Swedish language and describes mesophilic fermented milk that is known to have various functional benefits and the potential to improve human health [2,3].
Viili has a pleasant sharp taste and a good diacetyl aroma linked to a tough texture and can be cut easily with a spoon. Diacetyl is a major flavor compound in viili. The domestic fermented milk is typically consumed in meals, especially at breakfast, and is also used as a snack food among kids. This product is consumed either in plain form or sweetened with jam and raisins, or with fruit or cereals. A traditional way of eating viili is by mixing it with cinnamon and sugar [4]. The fat content in the product may vary from 2 to almost 12%, depending on classification (such as low fat, full fat). Viili was historically made on farms in large wooden buckets, and at a later stage, families also started to make it at home. The industrial manufacture of viili began in Finland in the 1950s. Today, this product has grown to be a vital fermented milk product in Finland. Annual consumption stands at more than 4.5 kg/capita [4]. The most traditional viili culture consists of mesophilic lactic acid bacteria (LAB) forming a culture in the body of the milk together with a surface-growing yeast- like fungus [4-8], thus all viili cultures also contain yeasts. The effect of yeasts on the viili is not clear, however, it is believed that yeasts in viili may provide the product’s unique flavor and induce the LAB to produce more exopolysaccharides (EPS) [7]. In this mini-review, we will shed a light on the manufacturing process of viili, including the identification and characteristics of microorganisms involved during culture. Moreover, biosynthesis of EPS and functional characteristics of viili are also discussed.
Identification of microorganisms in viili starter cultures
In the traditional manufacturing of viili, the propagation of the microflora was done by adding a small quantity of a previously prepared product. Today, the production of viili has developed to industrial-scale manufacturing; nevertheless, traditional starter cultures containing mixed populations of LAB are being utilized for this purpose. Four LAB strains, namely, the acid-producing strains Lactococcus lactis subsp. cremoris and L. lactis subsp. lactis, together with the aroma-producing strains Lactobacillus lactis subsp. lactis biovar. diacetylactis and Leuconostoc mesenteroides subsp. cremoris, were found. Wang et al. [7] identified two LAB strains, L. lactis subsp. cremoris and Leu. mesenteroides subsp. mesenteroides, in Taiwanese viili culture. On the other hand, Chen et al. [1] identified a different microbial profile in a viili culture, which included Lb. plantarum, Streptococcus thermophilus, Lb. paracasei, and Bacillus cereus. The strains other than L. lactis and Leu. Mesenteroides found in viili products, entered the culture from the local environment. Along with using the fungi (Geotrichum candidum), there are Kluyveromyces marxianus, Saccharomyces unisporus, and Pichiafermentans which has beenidentified in viili starter cultures at levels of 58%, 11%, and 31%, respectively. Viili yeasts produce carbon dioxide and alcohol, thus, contributing to the typical yeast flavor.
A steady and constant starter culture, which is necessary for manufacturing a quality fermented beverage, can be difficult to sustain due to the complex microbiological composition of viili culture. Therefore, knowledge of microbiological profiles of viili cultures is crucial to safety characteristics and quality control of viili products. Additionally, their probiotic effects need to be evaluated to determine their potential health benefits. Numerous studies have identified a variety of microorganisms in viili starter cultures using selective growth medium, morphological characterization, biochemical characterization, and molecular methods [1,4,7,9].
The characteristics of microorganisms of viili during culture
The fermentation of viili starters is characterized by a rapid increase in the number of LAB from an initial value of 106 to 109 CFU/g after the first 20 h of fermentation. This level then remains stable for the rest of process [7]. A study of the microbial dynamics at different fermentation stages demonstrated that L. Lactis subsp. cremoris was the leading bacterial species in the samples, followed by Leu. mesenteroides subsp. mesenteroides;moreover, there was no change in this proportion as the fermentation progressed. Uchida et al. [10] also reported that L. lactis subsp. cremoris was the dominant strain in all samples of Japanese domestic ropy fermented milk. A high population of L. lactis subsp. cremoris, which results in the production of a high level of EPS, is necessary to produce the ropiness of the fermented milk product. The viili fungus, G. candidum, contributes to the typical musty aroma of this produce. Lipases and proteases produced by G. candidum release fatty acids and peptides that are metabolized by the microbial populations, and this contributes to the development of viili’s distinctive flavors and other qualities [11]. Also, G. candidum neutralizes the curd by catabolizing the lactic acid produced by the LAB and by releasing ammonia during the metabolism of the amino acids [12]. The viili yeast, K. marxianus, is able to utilize both lactose and galactose as carbon sources, which explains why this strain is the primary yeast in the viili samples. The participation of K. marxianus in the starter culture ensures the metabolism of lactose via alcohol fermentation and the formation of the product’s typical yeasty flavor. In addition to K. marxianus, various lactose-negative yeasts seem to play very important roles in the formation of viili flavor. Simova et al. [13] reported that the product’s typical yeasty flavor and aroma were absent, when ropy fermented products were produced by K. marxianus only. Additionally, it is worthwhile mentioning that there are remarkable differences in the cell surface properties, co-aggregation, auto-aggregation, and biofilm formation between the kefir and viili strains [14]. The LAB and yeasts in viili do not show any significant auto-aggregation and biofilm formation, both of which are necessary for forming starter grains. Grain formation begins with the self-aggregation of LAB strains to form small granules. Biofilm producing strains then begin to attach to the surface of the granules and co-aggregatewith other organisms and components in the milk to form the grains [14]. The surface properties of viili microorganism explain why there is no starter grain formation in viili starter cultures.
Manufacture of viili at home
To make homemade viili (Figure 1), raw milk is pasteurized. Active starter (5%, w/v), usually from the previous day viili, is then inoculated into the milk, and the mixture is incubated at 20°C for 20 h. After fermentation, the viili is cooled to 4°C and is ready to consume. However, the ropy nature of viili products seems to become unstable if it is produced at higher fermentation temperatures and, when there are frequent transfers of starter cultures.
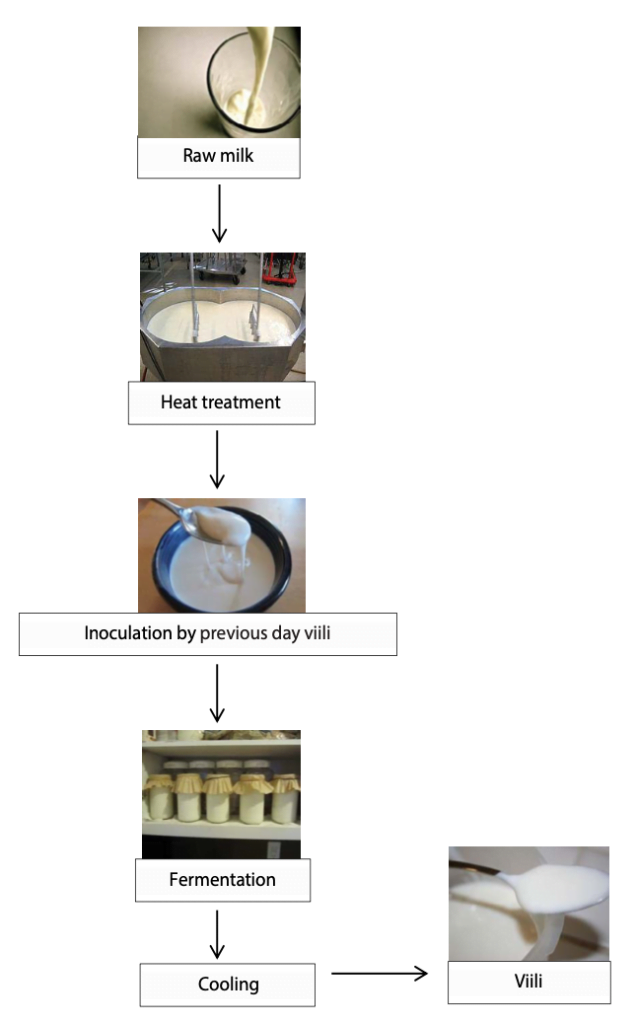
Figure 1. Manufacturing of viili at home.
Try our recipe
- 14 November 2023
- by Grondin Eric
How to make Viili at home?
Manufacture of viili in the factory
For the industrial production of viili (Figure 2), the milk is separated and standardized to a fat content in the range of 1.0%–3.5%. Usually, viili has been made from raw milk. Consequently, a layer of cream forms on its surface. After standardization, milk is heated to a high temperature (83°C for 20-25 min), tempered to the incubation temperature of 20°C, and inoculated with 4% starter culture consisting of L. lactis subsp. lactis, a diacetylactis culture, Leuc. mesenteroides subsp. cremoris, and Geotrichum candidum or a mixed culture of L. lactis subsp. cremoris, L. lactis subsp. lactis, and G. candidum. Next, the mixture is packed in a cup and removed to a storage area for ripening, where the viili fermentation takes place for 20 h at 20°C (final acidity of 0.9%). The purpose of incubation in consumer cups is to allow the fat to rise to the surface during incubation where the Geotrichum mold will grow and contribute to the typical musty aroma. Furthermore, complex carbohydrates formed by the organisms employed give the product a heavy and ropy characteristic. The viili is cooled to below 6°C after fermentation. The mold stops growing after the oxygen is completely consumed. Most of the CO2 formed dissolves in the product, leading to a slight underpressure in the package. The shelf life of viili is around 3 weeks. There is a wide range of different types of viili in the market, including products with dissimilar fat contents, oneswith reduced lactose and flavored varieties. Viili is also produced commercially from homogenized milk without mold growing on the surface and from milk other than cow’s, but traditional viili is still the most popular in the Finnish region [4,15].

Figure 2. Manufacturing of viili in the factory.
Slime properties of viili
The characteristic ropy consistency of viili is caused by the slime forming variants of L. lactis subsp. lactis and L. lactis subsp. Cremoris found in the starter culture [16]. Both strains are able to produce slime as capsule or exocellular EPS. Scanning electron micrographs of milk gels prepared using a ropy strain, L. Lactis subsp. cremoris, showed that EPS was present in the form of a network that attached the bacterial cells to the protein matrix. A thick network of slime also attached the casein micelle-clusters to each other producing casein conglomerates, which seems to be the origin of the characteristic consistency of viili [17].
Sugar components and composition of the EPS
The composition of the EPS excreted by L. lactis subsp. cremoris consists of 3-47% protein and 29-85% carbohydrate. The sugar components are predominantly galactose, glucose, and often rhamnose [18], with repeating units of “→4-β-glucopyranosyl-(1→4)- β-D-galactopyranosyl-(1 →4)-β-D- glucopyranosyl—(1→,” and groups of α-l-rhamnopyranosyl and α-d-galactopyranosyl-1-p attached to each side of galactopyranosyl [16,18,19]. The composition and sugar components of EPS are strain and medium dependent. The EPSs secreted by L. lactis subsp. cremoris SBT 0495, ARH53, ARH74, ARH 84, ARH 87, and B30 are composed of repeating units of galactose, glucose, and rhamnose with a phosphodiester structural element [18,20]. In contrast, the EPS produced by L. lactis subsp. cremoris H414 is a homopolymer consisting of galactose with a branched-pentasaccharide repeating unit [21].
Marshall et al. [22] reported that L. lactis subsp. cremoris strain LC33 was able to generate two different EPSs. One contains glucose, galactose, rhamnose, glucosamine, and phosphate. The other was composed of galactose, glucose, and glucosamine with branched terminal galactose moieties. The slime material obtained from L. lactis subsp. cremoris SBT0495 supernatant in whey permeate medium consisted of 42% carbohydrate and 21% protein with large amount of mannans present in the cultured medium [20]. Furthermore, L. lactis produced more EPS on glucose than on fructose as the sugar substrate, although the transcription level of the EPS gene cluster was independent of the sugar source [23].
Applications and biosynthesis of EPS
EPS synthesized by LAB is playing a major role in viili product and other fermented dairy products including cheese, drinking yoghurt, yoghurt, and fermented cream. EPS is increasing the viscosity of a final product and binding hydration water and interacting with other milk constituents, including proteins and micelles, to strengthen the rigidity of the casein network, thereby, improving the texture of final product. As a consequence, EPS can decrease syneresis and improve product stability. Furthermore, it has been conveyed that EPS has beneficial effects on gut health [24].
Recent studies have demonstrated that the ropy phenotype of L. Lactis subsp. cremoris is associated with an 18.5-30 MDa plasmid [23,25]. Several enzymes under positive or negative control are involved in polysaccharide synthesis and excretion. Threfore, the plasmid may code for certain proteins that are involved in slime production [26]. The metabolic pathways that are involved in the biosynthesis of the EPS molecules from the milk sugar lactose are illustrated in Figure 3. The biosynthesis can be roughly divided into three steps: the intracellular formation of EPS precursors, the sugar nucleotides, and the formation of a repeating unit on a lipid carrier that is located at the cytoplasmic membrane [23,27]. The repeating unit of EPS produced by most of L. lactis subsp. cremoris strains is composed of glucose, galactose, rhamnose, and phosphate [18,20]. The sugar nucleotides UDP- glucose, UDPgalactose, and dTDP-rhamnose are the donors of the monomers used for the biosynthesis of the pentasaccharide unit. The last step of EPS formation involves the transportation of the repeating units across the membrane to the outer layer of the bacteria and polymerization of several hundred to several thousand repeating units to form the final EPS [23,27]. The enzymes involved in EPS formation by L. lactis subsp. cremoris include the enzymes responsible for the original metabolism of the carbohydrate, enzymes leading to sugar nucleotide synthesis and interconversion, glycosyl transferases that form the repeating unit attached to the glycosyl carrier lipid, and translocases and polymerases that form the polymer. The genes encoding the enzymes involved in the biosynthesis of EPS by L. lactis subsp. cremoris are borne by an EPS plasmid. The gene products EpsD, EpsE, EpsF, and EpsG are glycosyltransferases and are required for the synthesis of the EPS backbone [28,29]. The presence of the EPS genes on a plasmid has been suggested to be the cause of EPS expression instability at higher temperatures and when there are frequent transfers of starter culture [30,31].
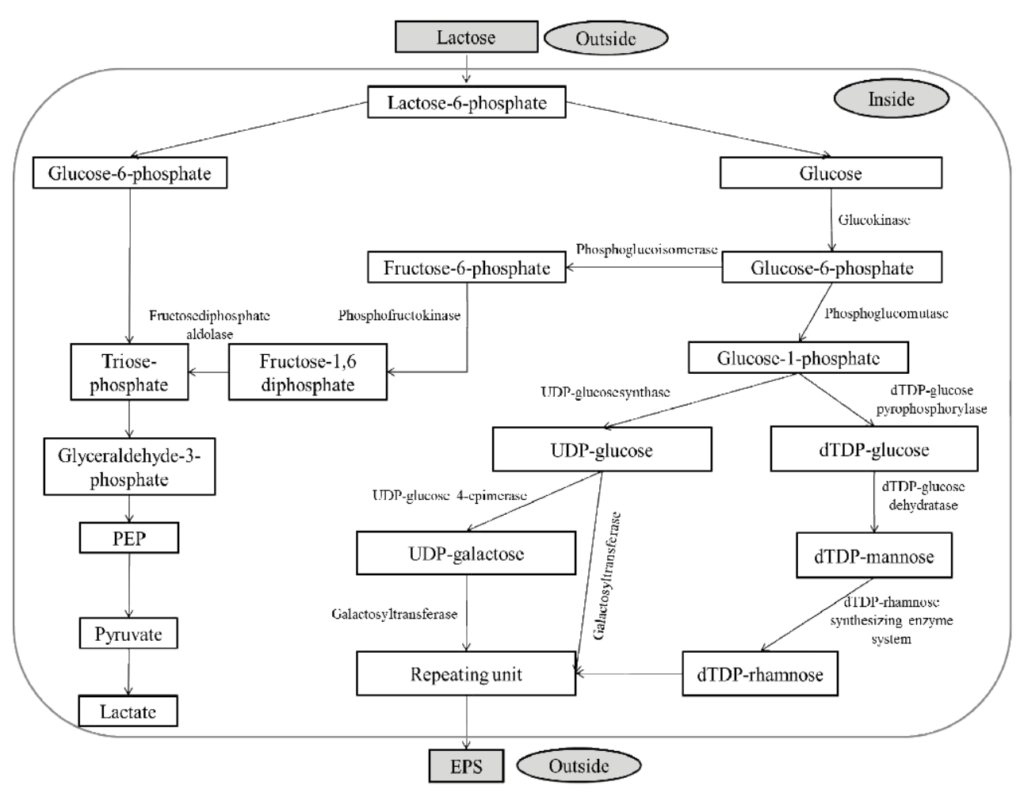
Figure 3. The biosynthesis of the EPS in L. lactis for EPS secretion.
Functional characteristics of viili
Viili and its isolated bacteria have been reported to possess several health benefits including anti-oxidation, ACE inhibitory, anti-tumor, and immunomodulatory activity.
Antioxidation activity: Endogenous metabolic processes and exogenous chemicals in the human body or in a food system are able to “in some circumstances” produce highly reactive oxygen species that are able to oxidize biomolecules, which results in tissue damage and cell death, thereby, leading to inflammation, diabetes, genotoxicity, cancer, and accelerated aging [32]. Viili made with viili bacteria have been demonstrated to containα,α-diphenyl-β-picylhydrazl, which has a free radical-scavenging effect and Fe2 + -chelating ability [2]. Viili EPS also possesses a strong antioxidant activity in the gastric intestine in vivo [33]. The antioxidative nature of viili and its products may help the human body to reduce oxidative damage. Several antioxidative peptides with free radical-scavenging activities have been identified in fermented dairy products [34-36]. Moreover, some studies have shown that certain L. lactis subsp. cremoris strains possess proteolytic abilities that aid the digestion of the milk protein into peptides and free amino acids [37,38]. The change in antioxidant activity noted in the low-fat cheeses made with viili is probably associated with the viable populations of LAB present in the cheese and the levels of antioxidative peptides.
Immunomodulatory activity: Viili and its LAB have been known to have immunoregulatory activates in vitro and in vivo, including antiallergic and anticolitis activates [39]. Viili and the viili bacterium L. lactis subsp. cremoris TL1 have been shown to induce the production of the helper cell type I (Th1) cytokine tumor necrosis factor-α, the proinflmmatory cytokine interleukin (IL)-6, and T regulatory cell (Treg) cytokine IL-10 in vitro, which suggests that viili may be helpful in improving the Th1/ Th2 balance. Oral feeding of the viili bacterium L. lactis subsp. cremoris TL1 has been shown to suppress total immunoglobulin IgE and ovalbumin (OVA)-specifi IgE levels in the serum of OVA-sensitized mice. Suppression of IgE production is an important target when treating allergies. Additionally, in vivo effcts of the viili bacterium L. lactis subsp. cremoris TL1 on the regulation of dextran sulfate sodium (DSS) intestinal physiology have been demonstrated. This strain is able to ameliorate DSS-induced colitis as exemplifid by a signifiant attenuation of the bleeding score and a reduction in colon shortening. Histological analysis also showed crypt regeneration and epithelial restitution in the colon among the animals in L. lactis subsp. cremoris TL1 treated group. These findings suggest that the viili isolated strain, L. lactis subsp. cremoris TL1, has a potential direct anti-inflammatory activity with respect to epithelial cells and that this may lead to inhibition of neutrophil accumulation in the mucosal region of the DSS-colitis mice.
ACE Inhibitory activity: ACE is known to associate with hypertension and congestive heart failure. Viili have been demonstrated to have a strong inhibitory effect on ACE activity [2]. The proteolytic activity of the starters and the rate of proteolysis both seem to play an important role in the inhibitory activity of these dairy products. The proteolytic system of L. lactis has been studied, which shows that the system consists of a cell wall–bound proteinase and several intracellular peptidases [37,38]. L. Lactis seems to possess high proteolytic activity, may produce a viili with better ACE inhibitory effect. Moreover, many studies have reported that the type and concentration of bioactive peptides present in a product are able to significantly affect the functional properties of the dairy product [40,41]. ACE inhibitory peptides are released from αs1-casein and β-caseins and also from α-lactalbumin and β-lactoglobulin during fermentation of milk by various microbiological cultures.
Antitumor activity: Studies have identified that viili possesses antitumor activity. Kitazawa et al. [42] reported that viili and one of the starter bacteria, L. lactis subsp. cremoris KVS20, exhibited antitumor activity against the metastasis of Lewis lung carcinoma, as well as acting to reduce the growth of solid and ascetic forms of sarcoma-180 in vivo. The antitumor effect of viili might be due to increase in the cytotoxic activity of the macrophages stimulated by L. lactis subsp. cremoris KVS20. Liu et al. [33] observed the senescence of HepG2 cancer cells after treatment with viili EPS, which supports the idea that viili EPS might have anti-tumor activity
Conclusion
Viili is a traditional fermented milk product involving LAB, as well as a surface mould which makes the product unique in taste, aroma and appearance compared to all other Nordic fermented milks. Viili is healthy food product which has beneficial effects on gut health, in addition to treatment many diseases such as cancer, inflammation, diabetes, genotoxicity, accelerated ageing, hypertension and heart diseases. Mostly, this is due to its functional activities including antioxidant, immunomodulatory, ACE Inhibitory and antitumor activity.
Chen TT, Tan QL, Wang MJ, Xiong SQ, Jiang SY, et al. (2011) Identification of bacterial strains in viili by molecular taxonomy and their synergistic effects on milk curd and exopolysaccharides production. Afr J Biotechnol 10: 16969-16975.
Chiang ML, Chen HC, Wang SY, Hsieh YL, Chen MJ (2011) Use of Taiwanese Ropy Fermented Milk (TRFM) and Lactococcus lactis subsp cremoris Isolated from TRFM in manufacturing of functional low-fat cheeses. J Food Sci 76: M504-M510.
Ruas-Madiedo P, Gueimonde M, de los Reyes-Gavilan CG, Salminen S (2006) Short communication: Effect of exopolysaccharide isolated from “viili” on the adhesion of probiotics and pathogens to intestinal mucus. J Dairy Sci 89: 2355-2358.
Leporanta K (2003) Viili and Långfil-Exotic fermented products from candinavia. Valio Foods and Functionals.
Boutrou R, Gueguen M (2005) Interests in Geotrichum candidum for cheese technology. Int J Food Microbiol 102: 1-20.
Kahala M, Maki M, Lehtovaara A, Tapanainen JM, Katiska R, et al. (2008) Characterization of starter lactic acid bacteria from the Finnish fermented milk product viili. J Appli Microbiol 105: 1929-1938.
Wang SY, Chen HC, Liu JR, Lin YC, Chen MJ (2008) Identification of yeasts and evaluation of their distribution in Taiwanese kefir and viili starters. J Dairy Sci 91: 3798-3805.
Wang Y, Liu Y, Luo C, Ding LC, Sun XN, et al. (2010) Initial isolation and identifiation of lactic acid bacteria in viili. J Anhui Agri Sci 38: 2831-2832.
Wang SY, Chen HC, Dai TY, Huang IN, Liu JR, et al. (2011) Identification of lactic acid bacteria in Taiwanese ropy fermented milk and evaluation of their microbial ecology in bovine and caprine milk. J Dairy Sci 94: 623-635.
Uchida K, Akashi K, Motoshima H, Urashima T, Arai I, et al. (2009) Microbiota analysis of Caspian Sea yogurt, a ropy fermented milk circulated in Japan. Animal Sci J 80: 187-192.
Holmquist M (1998) Insights into the molecular basis for fatty acyl specificities of lipases from Geotrichum candidum and Candida rugosa. Chem Phys Lipids 93: 57-66.
Greenberg RS, Ledford RA (1979) Deamination of glumatic and aspartic acids by Geotrichum candidum. J Dairy Sci 62: 368-372.
Simova E, Beshkova D, Angelov A, Hristozova T, Frengova G, et al. (2002) Lactic acid bacteria and yeasts in kefir grains and kefir made from them. J Ind Microbiol Biotechnol 28: 1-6.
Wang SY, Chen KN, Lo YM, Chiang ML, Chen HC, et al. (2012) Investigation of microorganisms involved in biosynthesis of the kefir grain. Food Microbiol 32: 274-285.
Tamime AY, Marshall VME (1997) Microbiology and technology of fermented milks. In: Law BA (ed.). Microbiology and biochemistry of cheese and fermented milk. Blackie Academic Co, London. pp: 57-152.
Nakajima H, Suzuki Y, Kaizu H, Hirota T (1992) Cholesterol lowering activity of ropy fermented milk. J Food Sci 57: 1327-1329.
Toba T, Nakajima H, Tobitani A, Adachi S (1990) Scanning electron microscopic and texture studies on characteristic consistency of Nordic ropy sour milk. Int J Food Microbiol 11: 313-320.
Nakajima H, Toyoda S, Toba T, Itoh T, Mukai T, et al. (1990) A novel phosphophlysaccharide from slime-forming Lactococcus lactis subspecies cremoris SBT 0495. J Dairy Sci 73: 1472-1477.
Sletmoen M, Maurstad G, Sikorski P, Paulsen BS, Stokke BT (2003) Characterisation of bacterial polysaccharides: steps towards single- molecular studies. Carbohydr Res 338: 2459-2475.
Yang ZN, Huttunen E, Staaf M, Widmalm G, Tenhu H (1999) Separation, purification and characterisation of extracellular polysaccharides produced by slime-forming Lactococcus lactis ssp cremoris strains. Int Dairy J 9: 631-638.
GruterM,LeeflangBR,KuiperJ,KamerlingJP,VliegenthartJF(1992) Structure of the exopolysaccharide produced by Lactococcus lactis subspecies cremoris H414 grown in a defined medium or skimmed milk. Carbohydr Res 231: 273-291.
MarshallVM,CowieEN,MoretonRS(1995)Analysisandproduction of two exopolysaccharides from Lactococcus lactis subsp. cremoris LC330. J Dairy Res 62: 621-628.
Looijesteijn PJ, Boels IC, Kleerebezem M, Hugenholtz J (1999) Regulation of exopolysaccharide production by Lactococcus lactis subsp. cremoris By the sugar source. Appl Environ Microbiol 65: 5003-5008.
Duboc P, Mollet B (2001) Applications of exopolysaccharides in the dairy industry. Int Dairy J 11: 759-768.
Knoshaug EP, Ahlgren JA, Trempy JE (2007) Exopolysaccharide expression in Lactococcus lactis subsp cremoris Ropy352: Evidence for novel gene organization. Appl Environ Microbiol 73: 897-905.
Kontusaari S, Forsén R (1988) Finnish fermented milk “Viili”: Involvement of two cell surface proteins in production of slime by Streptococcus lactis ssp. cremoris. J Dairy Sci 71: 3197-3202.
Hugenholtz J, Looijesteijn E, Starrenburg M, Dijkema C (2000) Analysis of sugar metabolism in an EPS producing Lactococcus lactis by P-31 NMR. J Biotechnol 77: 17-23.
van Kranenburg R, Marugg JD, van Swam II, Willem NJ, de Vos WM (1997) Molecular characterization of the plasmid-encoded eps gene cluster essential for exopolysaccharide biosynthesis in Lactococcus lactis. Mol Microbiol 24: 387-397.
van Kranenburg R, van Swam II, Marugg JD, Kleerebezem M, de Vos WM (1999) Exopolysaccharide biosynthesis in Lactococcus lactis NIZO B40: Functional analysis of the glycosyltransferase genes involved in synthesis of the polysaccharide backbone. J Bacteriol 181: 338-340.
Cerning J, Bouillanne C, Landon M, Desmazeaud M (1992) Isolation and characterization of exopolysaccharides from slime-forming mesophilic lactic-acid bacteria. J Dairy Sci 75: 692-699.
Vedamuthu ER, Neville JM (1986) Involvement of a Plasmid in Production of Ropiness (Mucoidness) in Milk Cultures by Streptococcus cremoris MS. Appl Environ Microbiol 51: 677-682.
Biswas M, Haldar PK, Ghosh AK (2010) Antioxidant and free-radical- scavenging effects of fruits of Dregea volubilis. J Nat Sci Biol Med 1: 29-34.
Liu L, Wu JH, Zhang JL, Li ZJ, Wang CL, et al. (2012) A compatibility assay of ursolic acid and foodborne microbial exopolysaccharides by antioxidant power and anti-proliferative properties in hepatocarcinoma cells. J Food Agri Environ 10: 111-114.
Hernandez-Ledesma B, Miralles B, Amigo L, Ramos M, Recio I (2005) Identification of antioxidant and ACE-inhibitory peptides in fermented milk. J Sci Food Agri 85: 1041-1048.
Kudoh Y, Matsuda S, Igoshi K, Oki T (2001) Antioxidative peptide from milk fermented with Lactobacillus delbrueckii subsp bulgaricus IFO13953. J Japanese Soci Food Sci Technol 48: 44-50.
Pritchard SR, Phillips M, Kailasapathy K (2010) Identification of bioactive peptides in commercial Cheddar cheese. Food Res Int 43: 1545-1548.
Alting AC, Engels WJM, Vanschalkwijk S, Exterkate FA (1995) Purification and characterization of cystathionine beta-lyase from lactococcus-lactis subsp, cremoris b78 and its possible role in flavor development in cheese. Appl Environ Microbiol 61: 4037-4042.
Tan PS, Konings WN (1990) Purification and characterization of an aminopeptidase from Lactococcus lactis subsp. cremoris Wg2. Appl Environ Microbiol 56: 526-532.
Huang IN, Dai TY, Wang SY, Chen MJ (2010) Inhibitory effect of Taiwanese ropy fermented milk in an ovalbumin-induced allergy mouse model. J Dairy Sci 93: 807-807.
OngL,ShahNP(2008)InfluenceofProbioticLactobacillusacidophilus and L-helveticus on proteolysis, organic acid profiles, and ACE- inhibitory activity of cheddar cheeses ripened at 4, 8, and 12 degrees C. J Food Sci 73: M111-M120.
Ong L, Shah NP (2008) Release and identification of angiotensin- converting enzyme-inhibitory peptides as influenced by ripening temperatures and probiotic adjuncts in Cheddar cheeses. Lwt-Food Sci Technol 41: 1555-1566.
Kitazawa H, Yamaguchi T, Itoh T (1992) B-cell mitogenic activity of slime products produced from slime-forming, encapsulated lactococcus-lactis ssp cremoris. J Dairy Sci 75: 2946-2951.
Leave a Comment