Fermented Beverage Benefits: A Comprehensive Review and Comparison of Kombucha and Kefir Microbiome
- 8 October 2023
- Grondin Eric
- (0)
- Kefir, Kombucha, Probiotics, Scientific paper
This blog article was written by the authors below and is shared in accordance with the Creative Commons CC BY license. No modifications have been made to its English version. If you choose the French language, an automatic translation by Google Translate is provided within the article.
Citation : Chong, A.Q.; Lau, S.W.; Chin, N.L.; Talib, R.A.; Basha, R.K. Fermented Beverage Benefits: A Comprehensive Review and Comparison of Kombucha and Kefir Microbiome. Microorganisms 2023, 11, 1344. https://doi.org/10.3390/microorganisms11051344
Copyright : © 2023 by the authors. Licensee MDPI, Basel, Switzerland. This article is an open access article distributed under the terms and conditions of the Creative Commons Attribution (CC BY) license (https://creativecommons.org/licenses/by/4.0/).
Abstract
Beverage fermentation is an ancient ritual that has been practised for millennia. It was slowly disappearing from households and communities due to the advancement of manufacturing technology and the marketing of soft drinks until the recent revival of the beverage fermentation culture due to an increase in the demand for health drinks amid the COVID-19 pandemic. Kombucha and kefir are two well-known fermented beverages that are renowned for their myriad of health benefits. The starter materials for making these beverages contain micro-organisms that act like microscopic factories producing beneficial nutrients that have antimicrobial and anticancer effects. The materials modulate the gut microbiota and promote positive effects on the gastrointestinal tract. Due to wide variations in the substrates and types of micro-organisms involved in the production of both kombucha and kefir, this paper compiles a compendium of the micro-organisms present and highlights their nutritional roles.
Keywords: kombucha; kefir; fermentation; micro-organisms; benefits; health
1. Introduction
Figure 1. Graphical abstract summarising comparisons between kombucha and kefir fermentation, factors affecting microbiome, and common benefits.
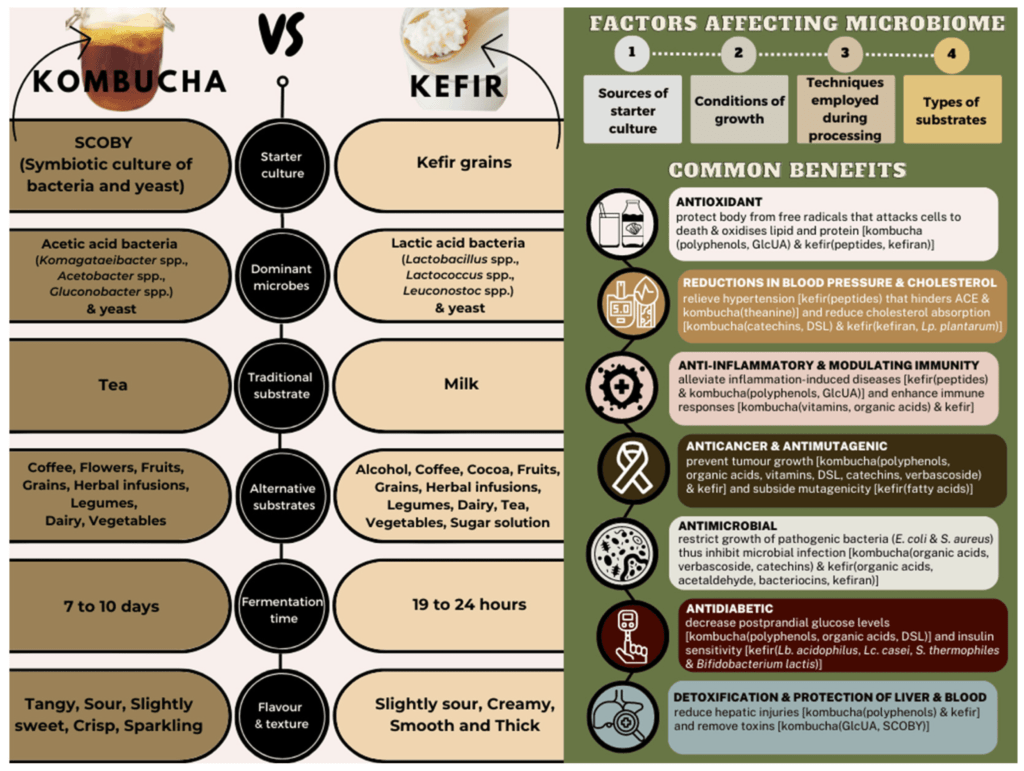
Table 1. Common benefits of kombucha and kefir.
2. Production of Kombucha and Kefir
Table 2. Substrates used in kombucha and kefir production.
The main pathways of substrate conversion into numerous products for both beverages were identified and are summarised in Figure 2. In kombucha fermentation, sucrose from the medium is first hydrolysed to simple sugars, namely glucose and fructose, by the enzyme invertase (β-fructofuranosidase, EC 3.2.1.26), which is primarily produced by yeast species, such as S. cerevisiae [5,169,170]. Yeasts synthesise ethanol and carbon dioxide as metabolites from the resultant monosaccharides, which is then oxidised by AAB to produce acetic acid over the following days [171,172]. The actual processes in kombucha are catalysed by the two primary metabolites, ethanol and acetic acid; acetic acid (most characteristic product of kombucha) promotes yeast to make ethanol, whereas ethanol stimulates the growth and production of AAB [5,18,155]. Concurrently, AAB are responsible for cellulose synthesis from glucose and fructose, which makes up the SCOBY [5,91,150,172]. Additionally, d-glucose at the C6 position and the aldehyde group of the β-d-glucose at the C1 position are both enzymatically oxidised by AAB, resulting in significant amounts of glucuronic acid (GlcUA) and d-glucano-δ-lactone, respectively [169]. This latter metabolite is hydrolysed into gluconic acid by microbial enzymes [169]. Other organic acids, such as oxalic, succinic, malic, and citric acids, play important roles in the biological processes by acting as intermediates or end products in metabolic pathways [96,173]. In some circumstances, metabolically active lactic acid bacteria (LAB) can produce a significant amount of lactic acid [174]. Apart from the main metabolites, chemical constituents present in kombucha originate from the substrate itself, where their structures can be altered and transformed into new components during fermentation [5]. With tea substrate, kombucha contains most of the tea ingredients, such as various polyphenols, flavonols, catechins, catechin gallates, adenine, caffeine, theobromine, theophylline, gallic acids, tannins, gallotannin, potassium, manganese, fluoride ions, vitamins A, B, C, E, and K, and amino acids, particularly theanine [5,18]. Vitamin C, the most common vitamin found in kombucha beverages, is assumed to be derived from glucose and synthesised by bacteria [96]. The changing profiles during the fermentation process and the end products are complex. The compositions depend on many factors, including the raw materials utilised, carbon source, amount of tea used, microbial makeup of the SCOBY, and conditions of fermentation process (time, temperature, and pH) [169].
Figure 2. Summary of the main pathways involved in kombucha and kefir fermentation.
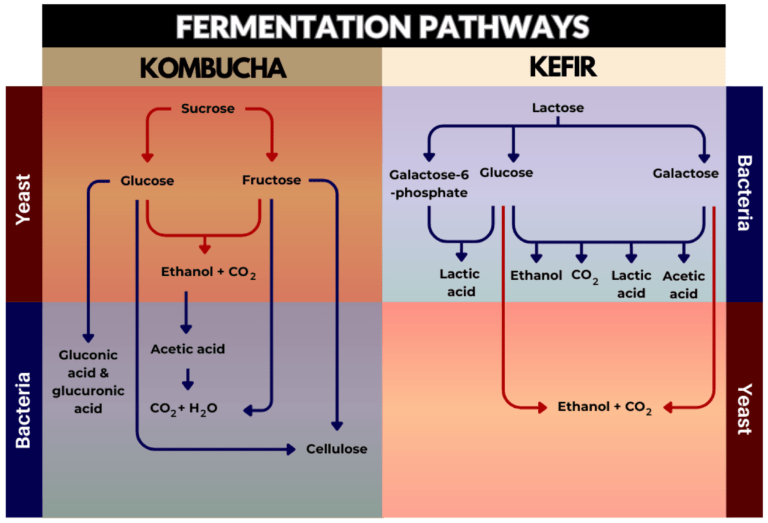
3. Microbial Communities
Table 3. Microbial composition in fermented kombucha produced using different substrates from various locations.
Abbreviations: A., Acetobacter; B., Bacterium; Brett., Brettanomyces; C., Candida; D., Dekkera; Gb., Gluconobacter; G., Gluconacetobacter; Kz., Kazachstania; Km., Kluyveromyces; Kb., Komagataeibacter; Lp., Lactiplantibacillus; Lh., Lachancea; Lq., Liquorilactobacillus; O., Oenococcus; P., Pichia; R., Rhodotorula; S., Saccharomyces; Sd., Saccharomycodes; Sz., Schizosaccharomyces; Sg., Sphigomonas; Tc., Tanticharoemia; T., Torulospora; Wm., Wickerhamomyces; Z., Zygosaccharomyces; Zt., Zygotorulaspora; Zm., Zymomonas.
Table 4. Microbial composition in fermented kefir produced using different substrates from various locations.
Abbreviations: A., Acetobacter; C., Candida; Gb., Gluconobacter; Kz., Kazachstania; Km., Kluyveromyces; Kb., Komagataeibacter; Lh., Lachancea; Lc., Lacticaseibacillus; Lp., Lactiplantibacillus; Lb., Lactobacillus; L., Lactococcus; Lt., Lentilactobacillus; Lv., Levilactobacillus; Leu., Leuconostoc; Lm., Limosilactobacillus; Lq., Liquorilactobacillus; M., Meyerozyma; P., Pichia; S., Saccharomyces; Sb., Schleiferilactobacillus; Sc., Streptococcus; T., Torulospora; Zt., Zygotorulaspora.
4. Health Benefits
4.1. Antioxidant
4.2. Reductions in Blood Pressure and Cholesterol
4.3. Anti-Inflammatory and Modulating Immunity
4.4. Anticancer and Antimutagenic
4.5. Antimicrobial
4.6. Antidiabetic
4.7. Detoxification and Protection of Liver and Blood
5. Future Perspectives of Kombucha and Kefir
The popularity of kombucha and kefir, along with the demand for their functional properties, has led to an increase in their production and, subsequently, the proliferation of starter cultures. The reusable and adaptive features of the cultures could serve as viable alternatives in various industries, including in the production of probiotic-rich foods and natural preservatives as well as packaging and waste management, potentially generating economic and sustainable benefits. Among the innovative options, one explorable approach is the use of diverse substrates. Comparing the substrates used in kombucha and kefir, it is evident that some substrates are unique to each of them. These unique substrates present an opportunity for further exploration into how they impact the microbial communities and chemical compositions of these drinks. While sugar initiates kefir fermentation, the exact process of kefir grain formation and the role of other substrate components remain unclear. Further elucidation of the metabolic pathways is therefore essential to understand the underlying mechanisms of fermentation and identify opportunities for process optimisation. Although the formation of SCOBY in kombucha is well understood, i.e., formed from cellulose produced by acetic acid bacteria and yeast, the exact nutrient source for optimal SCOBY growth has not been well defined. More research is needed to understand the SCOBY needs and its relationship with microbial diversity in kombucha. Despite the successful identification of some micro-organisms present in kombucha and kefir, there is still a considerable research gap in understanding how these microbial communities specifically contribute to health and the sensory characteristics of these drinks, particularly the impact on the overall drinking experience. As such, further research is necessary to better understand the interactions among substrates, microbial communities, and human physiology in order to optimise the health benefits and sensory qualities of these drinks.
6. Conclusions
Fermentation has brought about the creation of functional beverages, such as kombucha and kefir, which share a common feature of being produced by the action of starter cultures, namely SCOBY and kefir grains, respectively, in a sugar-containing liquid. In kombucha, the dominant micro-organisms are acetic acid bacteria and yeast, while in kefir, lactic acid bacteria and yeast play the dominant roles in the fermentation process. The microbial communities of these functional beverages are significantly different based on the substrates and origins of the starter culture used, resulting in overlapping yet distinct health benefits. Both beverages contain probiotics and polyphenols, which scavenge free radicals and protect the body from oxidative attacks, which may help prevent hypertension and atherosclerosis. Bioactive peptides, GIcUA, and catechins are some of the metabolites that may act as immunity modulators. Kombucha and kefir display anticancer properties, contributed by catechins and verbascoside in kombucha and antimutagenic components in kefir. These beverages may also protect the body from microbial attacks and have shown their potential in controlling diabetic conditions and liver problems. Addressing the challenges and limitations through ongoing research is crucial for recognising the potential benefits of kombucha and kefir. This could ultimately lead to advancements in the field, promoting longevity and well-being.
Writing—original draft preparation, A.Q.C. and S.W.L.; writing—review and editing, N.L.C.; supervision, N.L.C., R.A.T. and R.K.B. All authors have read and agreed to the published version of the manuscript.
This research received no external funding.
No new data were created or analysed in this study. Data sharing is not applicable to this article.
The authors declare no conflict of interest.
- Allaith, S.A.; Abdel-Aziz, M.E.; Thabit, Z.A.; Altemimi, A.B.; El-Ghany, K.A.; Giuffrè, A.M.; Al-Manhel, A.J.A.; Ebrahim, H.S.; Mohamed, R.M.; Abedelmaksoud, T.G. Screening and Molecular Identification of Lactic Acid Bacteria Producing β-Glucan in Boza and Cider. Fermentation 2022, 8, 350. [Google Scholar] [CrossRef]
- Osipova, M.V. Use of Clarifying Agents in Technological Process of Kvass Production. IOP Conf. Ser. Earth Environ. Sci. 2020, 613, 012100. [Google Scholar] [CrossRef]
- Iglesias, A.; Pascoal, A.; Choupina, A.B.; Carvalho, C.A.; Feás, X.; Estevinho, L.M. Developments in the Fermentation Process and Quality Improvement Strategies for Mead Production. Molecules 2014, 19, 12577–12590. [Google Scholar] [CrossRef]
- Kanamoto, E.; Terashima, K.; Shiraki, Y.; Nishida, H. Diversity of Bacillus Isolates from the Sake Brewing Process at a Sake Brewery. Microorganisms 2021, 9, 1760. [Google Scholar] [CrossRef] [PubMed]
- de Miranda, J.F.; Ruiz, L.F.; Silva, C.B.; Uekane, T.M.; Silva, K.A.; Gonzalez, A.G.M.; Fernandes, F.F.; Lima, A.R. Kombucha: A Review of Substrates, Regulations, Composition, and Biological Properties. J. Food Sci. 2022, 87, 503–527. [Google Scholar] [CrossRef] [PubMed]
- Silva, K.A.; Uekane, T.M.; de Miranda, J.F.; Ruiz, L.F.; da Motta, J.C.B.; Silva, C.B.; Pitangui, N.D.S.; Gonzalez, A.G.M.; Fernandes, F.F.; Lima, A.R. Kombucha Beverage from Non-Conventional Edible Plant Infusion and Green Tea: Characterization, Toxicity, Antioxidant Activities and Antimicrobial Properties. Biocatal. Agric. Biotechnol. 2021, 34, 102032. [Google Scholar] [CrossRef]
- Greenwalt, C.J.; Steinkraus, K.H.; Ledford, R.A. Kombucha, the Fermented Tea: Microbiology, Composition, and Claimed Health Effects. J. Food Prot. 2000, 63, 976–981. [Google Scholar] [CrossRef]
- Kim, J.; Adhikari, K. Current Trends in Kombucha: Marketing Perspectives and the Need for Improved Sensory Research. Beverages 2020, 6, 15. [Google Scholar] [CrossRef]
- Ganatsios, V.; Nigam, P.; Plessas, S.; Terpou, A. Kefir as a Functional Beverage Gaining Momentum towards Its Health Promoting Attributes. Beverages 2021, 7, 48. [Google Scholar] [CrossRef]
- Lopitz-Otsoa, F.; Rementeria, A.; Elguezabal, N.; Garaizar, J. Kefir: Una comunidad simbiótica de bacterias y levaduras con propiedades saludables. Rev. Iberoam. De Micol. 2006, 23, 67–74. [Google Scholar] [CrossRef]
- Nyhan, L.M.; Lynch, K.M.; Sahin, A.W.; Arendt, E.K. Advances in Kombucha Tea Fermentation: A Review. Appl. Microbiol. 2022, 2, 73–103. [Google Scholar] [CrossRef]
- Ozer, B.; Kirmaci, H. Fermented milks: Products of eastern Europe and Asia. In Encyclopedia of Food Microbiology; Academic Press: Cambridge, MA, USA, 2014. [Google Scholar] [CrossRef]
- Hasler, C.M. Functional Foods: Benefits, Concerns and Challenges—A Position Paper from the American Council on Science and Health. J. Nutr. 2002, 132, 3772–3781. [Google Scholar] [CrossRef] [PubMed]
- Kombucha Market Size, Share & COVID-19 Impact Analysis, By Type (Natural and Flavored), Distribution Channel (Supermarkets/Hypermarkets, Convenience Stores, Health Stores, and Online Retail), and Regional Forecast, 2022–2027). Available online: https://www.fortunebusinessinsights.com/industry-reports/kombucha-market-100230 (accessed on 10 February 2023).
- Kefir Market Size, Share & COVID-19 Impact Analysis, By Product Type (Dairy-Based & Non-Dairy), By Nature (Organic & Conventional), Category (Flavored & Non-Flavored), Distribution Channel (Supermarkets/Hypermarkets, Convenience Stores, Specialty Stores, & Online Retail), and Regional Forecast, 2020–2027. Available online: https://www.fortunebusinessinsights.com/kefir-market-102463 (accessed on 10 May 2023).
- Simova, E.; Beshkova, D.; Angelov, A.; Hristozova, T.; Frengova, G.; Spasov, Z. Lactic Acid Bacteria and Yeasts in Kefir Grains and Kefir Made from Them. J. Ind. Microbiol. Biotechnol. 2002, 28, 1–6. [Google Scholar] [CrossRef] [PubMed]
- Tamime, A. Fermented Milks; Tamime, A., Ed.; Blackwell Publishing Ltd.: Oxford, UK, 2006; ISBN 9780470995501. [Google Scholar]
- Lončar, E.; Djurić, M.; Malbaša, R.; Kolarov, L.; Klašnja, M. Influence of Working Conditions Upon Kombucha Conducted Fermentation of Black Tea. Food Bioprod. Process 2006, 84, 186–192. [Google Scholar] [CrossRef]
- Assadi, M.; Pourahmad, R.; Moazami, N. Use of Isolated Kefir Starter Cultures in Kefir Production. World J. Microbiol. Biotechnol. 2000, 16, 541–543. [Google Scholar] [CrossRef]
- Ahmed, R.F.; Hikal, M.S.; Abou-Taleb, K.A. Biological, Chemical and Antioxidant Activities of Different Types Kombucha. Ann. Agric. Sci. 2020, 65, 35–41. [Google Scholar] [CrossRef]
- Cardoso, R.R.; Neto, R.O.; Dos Santos D’Almeida, C.T.; Nascimento, T.; Pressete, C.G.; Azevedo, L.; Martino, H.S.D.; Cameron, L.C.; Ferreira, M.S.L.; de Barros, F.A.R. Kombuchas from Green and Black Teas Have Different Phenolic Profile, Which Impacts Their Antioxidant Capacities, Antibacterial and Antiproliferative Activities. Food Res. Int. 2020, 128, 108782. [Google Scholar] [CrossRef]
- Chu, S.-C.; Chen, C. Effects of Origins and Fermentation Time on the Antioxidant Activities of Kombucha. Food Chem. 2006, 98, 502–507. [Google Scholar] [CrossRef]
- Fu, C.; Yan, F.; Cao, Z.; Xie, F.; Lin, J. Antioxidant Activities of Kombucha Prepared from Three Different Substrates and Changes in Content of Probiotics during Storage. Food Sci. Technol. 2014, 34, 123–126. [Google Scholar] [CrossRef]
- Jayabalan, R.; Subathradevi, P.; Marimuthu, S.; Sathishkumar, M.; Swaminathan, K. Changes in Free-Radical Scavenging Ability of Kombucha Tea during Fermentation. Food Chem. 2008, 109, 227–234. [Google Scholar] [CrossRef]
- Kallel, L.; Desseaux, V.; Hamdi, M.; Stocker, P.; Ajandouz, E.H. Insights into the Fermentation Biochemistry of Kombucha Teas and Potential Impacts of Kombucha Drinking on Starch Digestion. Food Res. Int. 2012, 49, 226–232. [Google Scholar] [CrossRef]
- Malbaša, R.V.; Lončar, E.S.; Vitas, J.S.; Čanadanović-Brunet, J.M. Influence of Starter Cultures on the Antioxidant Activity of Kombucha Beverage. Food Chem. 2011, 127, 1727–1731. [Google Scholar] [CrossRef]
- Srihari, T.; Arunkumar, R.; Arunakaran, J.; Satyanarayana, U. Downregulation of Signalling Molecules Involved in Angiogenesis of Prostate Cancer Cell Line (PC-3) by Kombucha (Lyophilized). Biomed. Prev. Nutr. 2013, 3, 53–58. [Google Scholar] [CrossRef]
- Sun, T.-Y.; Li, J.-S.; Chen, C. Effects of Blending Wheatgrass Juice on Enhancing Phenolic Compounds and Antioxidant Activities of Traditional Kombucha Beverage. J. Food Drug Anal. 2015, 23, 709–718. [Google Scholar] [CrossRef]
- Liu, J.-R.; Chen, M.-J.; Lin, C.-W. Antimutagenic and Antioxidant Properties of Milk−Kefir and Soymilk−Kefir. J. Agric. Food Chem. 2005, 53, 2467–2474. [Google Scholar] [CrossRef] [PubMed]
- Liu, J.-R.; Lin, Y.-Y.; Chen, M.-J.; Chen, L.-J.; Lin, C.-W. Antioxidative Activities of Kefir. Asian Australas. J. Anim. Sci. 2005, 18, 567–573. [Google Scholar] [CrossRef]
- Guven, A.; Gulmez, M. The Effect of Kefir on the Activities of GSH-Px, GST, CAT, GSH and LPO Levels in Carbon Tetrachloride-Induced Mice Tissues. J. Veter. Med. Ser. B 2003, 50, 412–416. [Google Scholar] [CrossRef]
- Ozcan, T.; Sahin, S.; Akpinar-Bayizit, A.; Yilmaz-Ersan, L. Assessment of Antioxidant Capacity by Method Comparison and Amino Acid Characterisation in Buffalo Milk Kefir. Int. J. Dairy Technol. 2018, 72, 65–73. [Google Scholar] [CrossRef]
- Karagozlu, C.; Unal, G.; Akalin, A.S.; Akan, E.; Kinik, O. The Effects of Black and Green Tea on Antioxidant Activity and Sensory Characteristics of Kefir. Agro. Food Ind. Hi. Tech. 2017, 28, 77–80. [Google Scholar]
- Patil, S.; Pimpley, V.; Warudkar, K.; Murthy, P.S. Valorisation of Coffee Pulp for Development of Innovative Probiotic Beverage Using Kefir: Physicochemical, Antioxidant, Sensory Analysis and Shelf Life Studies. Waste Biomass Valorization 2021, 13, 905–916. [Google Scholar] [CrossRef]
- Yilmaz-Ersan, L.; Ozcan, T.; Akpinar-Bayizit, A.; Sahin, S. The Antioxidative Capacity of Kefir Produced from Goat Milk. Int. J. Chem. Eng. Appl. 2016, 7, 22–26. [Google Scholar] [CrossRef]
- Dufresne, C.; Farnworth, E. Tea, Kombucha, and health: A review. Food Res. Int. 2000, 33, 409–421. [Google Scholar] [CrossRef]
- Martínez Leal, J.; Valenzuela Suárez, L.; Jayabalan, R.; Huerta Oros, J.; Escalante-Aburto, A. A Review on Health Benefits of Kombucha Nutritional Compounds and Metabolites. CyTA J. Food 2018, 16, 390–399. [Google Scholar] [CrossRef]
- Yang, Z.-W.; Ji, B.-P.; Zhou, F.; Li, B.; Luo, Y.; Yang, L.; Li, T. Hypocholesterolaemic and Antioxidant Effects of Kombucha Tea in High-Cholesterol Fed Mice. J. Sci. Food Agric. 2008, 89, 150–156. [Google Scholar] [CrossRef]
- Möller, N.P.; Scholz-Ahrens, K.E.; Roos, N.; Schrezenmeir, J. Bioactive Peptides and Proteins from Foods: Indication for Health Effects. Eur. J. Nutr. 2008, 47, 171–182. [Google Scholar] [CrossRef] [PubMed]
- Maeda, H.; Zhu, X.; Suzuki, S.; Suzuki, K.; Kitamura, S. Structural Characterization and Biological Activities of an Exopolysaccharide Kefiran Produced by Lactobacillus kefiranofaciens WT-2BT. J. Agric. Food Chem. 2004, 52, 5533–5538. [Google Scholar] [CrossRef]
- Liu, J.-R.; Wang, S.-Y.; Chen, M.-J.; Chen, H.-L.; Yueh, P.-Y.; Lin, C.-W. Hypocholesterolaemic Effects of Milk-Kefir and Soyamilk-Kefir in Cholesterol-Fed Hamsters. Br. J. Nutr. 2006, 95, 939–946. [Google Scholar] [CrossRef]
- Guzel-Seydim, Z.B.; Kok-Tas, T.; Greene, A.K.; Seydim, A.C. Review: Functional Properties of Kefir. Crit. Rev. Food Sci. Nutr. 2011, 51, 261–268. [Google Scholar] [CrossRef]
- Vujičić, I.F.; Vulić, M.; Könyves, T. Assimilation of Cholesterol in Milk by Kefir Cultures. Biotechnol. Lett. 1992, 14, 847–850. [Google Scholar] [CrossRef]
- Wang, Y.; Xu, N.; Xi, A.; Ahmed, Z.; Zhang, B.; Bai, X. Effects of Lactobacillus plantarum MA2 Isolated from Tibet Kefir on Lipid Metabolism and Intestinal Microflora of Rats Fed on High-Cholesterol Diet. Appl. Microbiol. Biotechnol. 2009, 84, 341–347. [Google Scholar] [CrossRef]
- Vázquez-Cabral, B.; Larrosa-Pérez, M.; Gallegos-Infante, J.; Moreno-Jiménez, M.; González-Laredo, R.; Rutiaga-Quiñones, J.; Gamboa-Gómez, C.; Rocha-Guzmán, N. Oak Kombucha Protects against Oxidative Stress and Inflammatory Processes. Chem. Interactions 2017, 272, 1–9. [Google Scholar] [CrossRef] [PubMed]
- Guruvayoorappan, C.; Kuttan, G. (+)−Catechin Inhibits Tumour Angiogenesis and Regulates the Production of Nitric Oxide and TNF-A in LPS-Stimulated Macrophages. J. Endotoxin Res. 2008, 14, 160–174. [Google Scholar] [CrossRef] [PubMed]
- Vina, I.; Semjonovs, P.; Linde, R.; Denina, I. Current Evidence on Physiological Activity and Expected Health Effects of Kombucha Fermented Beverage. J. Med. Food 2014, 17, 179–188. [Google Scholar] [CrossRef] [PubMed]
- Sinir, G.Ö.; Tamer, C.E.; Suna, S. Kombucha Tea: A Promising Fermented Functional Beverage. In Fermented Beverages; Elsevier: Amsterdam, The Netherlands, 2019; Volume 5, pp. 401–432. [Google Scholar]
- Vinderola, G.; Perdigón, G.; Duarte, J.; Farnworth, E.; Matar, C. Effects of the Oral Administration of the Products Derived from Milk Fermentation by Kefir Microflora on Immune Stimulation. J. Dairy Res. 2006, 73, 472–479. [Google Scholar] [CrossRef] [PubMed]
- Vinderola, C.G.; Duarte, J.; Thangavel, D.; Perdigón, G.; Farnworth, E.; Matar, C. Immunomodulating Capacity of Kefir. J. Dairy Res. 2005, 72, 195–202. [Google Scholar] [CrossRef]
- Thoreux, K.; Schmucker, D.L. Kefir Milk Enhances Intestinal Immunity in Young but Not Old Rats. J. Nutr. 2001, 131, 807–812. [Google Scholar] [CrossRef]
- Deghrigue, M.; Chriaa, J.; Battikh, H.; Abid, K.; Bakhrouf, A. Antiproliferative and Antimicrobial Activities of Kombucha Tea. Afr. J. Microbiol. Res. 2013, 7, 3466–3470. [Google Scholar]
- Yang, C.S.; Wang, H. Cancer Preventive Activities of Tea Catechins. Molecules 2016, 21, 1679. [Google Scholar] [CrossRef]
- Zhang, Y.; Yuan, Y.; Wu, H.; Xie, Z.; Wu, Y.; Song, X.; Wang, J.; Shu, W.; Xu, J.; Liu, B.; et al. Effect of Verbascoside on Apoptosis and Metastasis in Human Oral Squamous Cell Carcinoma. Int. J. Cancer 2018, 143, 980–991. [Google Scholar] [CrossRef]
- Güzel-Seydim, Z.B.; Seydim, A.C.; Greene, A.K.; Taş, T. Determination of Antimutagenic Properties of Acetone Extracted Fermented Milks and Changes in Their Total Fatty Acid Profiles including Conjugated Linoleic Acids. Int. J. Dairy Technol. 2006, 59, 209–215. [Google Scholar] [CrossRef]
- De Moreno De LeBlanc, A.; Matar, C.; Farnworth, E.; Perdigón, G. Study of Immune Cells Involved in the Antitumor Effect of Kefir in a Murine Breast Cancer Model. J. Dairy Sci. 2007, 90, 1920–1928. [Google Scholar] [CrossRef] [PubMed]
- Liu, J.-R.; Wang, S.-Y.; Lin, Y.-Y.; Lin, C.-W. Antitumor Activity of Milk Kefir and Soy Milk Kefir in Tumor-Bearing Mice. Nutr. Cancer 2002, 44, 183–187. [Google Scholar] [CrossRef] [PubMed]
- Sozmen, M.; Erginsoy, S.D.; Cenesiz, S.; Devrim, A.K. The Protective Effect of Kefir and Vitamin C on Azoxymethane Induced Toxicity and Induction of Metallothionein in Mice. Scand. J. Lab. Anim. Sci. 2005, 32, 211–220. [Google Scholar]
- Cenesiz, S.; Devrima, K.; Kamber, U.; Sozmen, M. DTW-The Effect of Kefir on Glutathione (GSH), Malondialdehyde (MDA) and Nitric Oxide (NO) Levels in Mice with Colonic Abnormal. DTW Dtsch. Tierarztl. Wochenschr. 2008, 115, 15–19. [Google Scholar] [CrossRef] [PubMed]
- Al-Mohammadi, A.-R.; Ismaiel, A.A.; Ibrahim, R.A.; Moustafa, A.H.; Zeid, A.A.; Enan, G. Chemical Constitution and Antimicrobial Activity of Kombucha Fermented Beverage. Molecules 2021, 26, 5026. [Google Scholar] [CrossRef] [PubMed]
- Shahbazi, H.; Gahruie, H.H.; Golmakani, M.-T.; Eskandari, M.H.; Movahedi, M. Effect of Medicinal Plant Type and Concentration on Physicochemical, Antioxidant, Antimicrobial, and Sensorial Properties of Kombucha. Food Sci. Nutr. 2018, 6, 2568–2577. [Google Scholar] [CrossRef] [PubMed]
- Ayed, L.; Ben Abid, S.; Hamdi, M. Development of a Beverage from Red Grape Juice Fermented with the Kombucha Consortium. Ann. Microbiol. 2017, 67, 111–121. [Google Scholar] [CrossRef]
- Ulusoy, B.H.; Çolak, H.; Hampikyan, H.; Erkan, M.E. An in Vitro Study on the Antibacterial Effect of Kefir against Some Food-Borne Pathogens. Vet. Med. 2007, 37, 103–107. [Google Scholar]
- Santos, A.; San Mauro, M.S.; Sanchez, A.; Torres, J.M.; Marquina, D. The Antimicrobial Properties of Different Strains of Lactobacillus spp. Isolated from Kefir. Syst. Appl. Microbiol. 2003, 26, 434–437. [Google Scholar] [CrossRef]
- Kakisu, E.J.; Abraham, A.G.; Pérez, P.F.; De Antoni, G.L. Inhibition of Bacillus cereus in Milk Fermented with Kefir Grains. J. Food Prot. 2007, 70, 2613–2616. [Google Scholar] [CrossRef]
- Medrano, M. Kefiran Antagonizes Cytopathic Effects of Bacillus cereus Extracellular Factors. Int. J. Food Microbiol. 2008, 122, 1–7. [Google Scholar] [CrossRef] [PubMed]
- Powell, J.; Witthuhn, R.; Todorov, S.; Dicks, L. Characterization of Bacteriocin ST8KF Produced by a Kefir Isolate Lactobacillus plantarum ST8KF. Int. Dairy J. 2007, 17, 190–198. [Google Scholar] [CrossRef]
- Aloulou, A.; Hamden, K.; Elloumi, D.; Ali, M.B.; Hargafi, K.; Jaouadi, B.; Ayadi, F.; Elfeki, A.; Ammar, E. Hypoglycemic and Antilipidemic Properties of Kombucha Tea in Alloxan-Induced Diabetic Rats. BMC Complement. Altern. Med. 2012, 12, 63. [Google Scholar] [CrossRef]
- Tanaka, T.; Kouno, I. Oxidation of Tea Catechins: Chemical Structures and Reaction Mechanism. Food Sci. Technol. Res. 2003, 9, 128–133. [Google Scholar] [CrossRef]
- Hosseini, S.A.; Rasouli, L.; Gorjian, M.; Yadollahpour, A. A Comparative Study of the Effect of Kombucha Prepared from Green and Black Teas on the Level of Blood Glucose and Lipid Profile of Diabetic Rats. Int. J. Pharm. Res. Allied Sci. 2016, 5, 93–102. [Google Scholar]
- Bhattacharya, S.; Gachhui, R.; Sil, P.C. The Prophylactic Role of D-Saccharic Acid-1,4-Lactone against Hyperglycemia-Induced Hepatic Apoptosis via Inhibition of Both Extrinsic and Intrinsic Pathways in Diabetic Rats. Food Funct. 2012, 4, 283–296. [Google Scholar] [CrossRef] [PubMed]
- Alihosseini, N.; Moahboob, S.; Farrin, N.; Mobasseri, M.; Taghizadeh, A.; Ostadrahimi, A. Effect of Probiotic Fermented Milk (Kefir) on Serum Level of Insulin and Homocysteine in Type 2 Diabetes Patients. Acta Endocrinol. (Bucharest) 2017, 13, 431–436. [Google Scholar] [CrossRef] [PubMed]
- Ostadrahimi, A.; Taghizadeh, A.; Mobasseri, M.; Farrin, N.; Payahoo, L.; Beyramalipoor Gheshlaghi, Z.B.; Vahedjabbari, M. Effect of Probiotic Fermented Milk (Kefir) on Glycemic Control and Lipid Profile in Type 2 Diabetic Patients: A Randomized Double-Blind Placebo-Controlled Clinical Trial. Iran. J. Public Health 2015, 44, 228–237. [Google Scholar]
- Kabiri, N.; Setorki, M.; Darabi, M.A. Protective Effects of Kombucha Tea and Silimarin against Thioacetamide Induced Hepatic Injuries in Wistar Rats. World Appl. Sci. J. 2013, 27, 524–532. [Google Scholar]
- Nguyen, N.K.; Nguyen, P.B.; Nguyen, H.T.; Le, P.H. Screening the Optimal Ratio of Symbiosis between Isolated Yeast and Acetic Acid Bacteria Strain from Traditional Kombucha for High-Level Production of Glucuronic Acid. LWT Food Sci. Technol. 2015, 64, 1149–1155. [Google Scholar] [CrossRef]
- Vīna, I.; Linde, R.; Patetko, A.; Semjonovs, P. Glucuronic Acid from Fermented Beverages: Biochemical Functions in Humans and Its Role in Health Protection. Int. J. Res. Rev. Appl. Sci. 2013, 14, 217–230. [Google Scholar]
- Ismaiel, A.A.; Bassyouni, R.H.; Kamel, Z.; Gabr, S.M. Detoxification of Patulin by Kombucha Tea Culture. CyTA J. Food 2015, 14, 271–279. [Google Scholar] [CrossRef]
- Chen, H.-L.; Tung, Y.-T.; Tsai, C.-L.; Lai, C.-W.; Lai, Z.-L.; Tsai, H.-C.; Lin, Y.-L.; Wang, C.-H.; Chen, C.-M. Kefir Improves Fatty Liver Syndrome by Inhibiting the Lipogenesis Pathway in Leptin-Deficient Ob/Ob Knockout Mice. Int. J. Obes. 2013, 38, 1172–1179. [Google Scholar] [CrossRef] [PubMed]
- Harrison, K.; Curtin, C. Microbial Composition of SCOBY Starter Cultures Used by Commercial Kombucha Brewers in North America. Microorganisms 2021, 9, 1060. [Google Scholar] [CrossRef]
- Laavanya, D.; Shirkole, S.; Balasubramanian, P. Current Challenges, Applications and Future Perspectives of SCOBY Cellulose of Kombucha Fermentation. J. Clean. Prod. 2021, 295, 126454. [Google Scholar] [CrossRef]
- Malbaša, R.; Lončar, E.; Djurić, M. Comparison of the Products of Kombucha Fermentation on Sucrose and Molasses. Food Chem. 2008, 106, 1039–1045. [Google Scholar] [CrossRef]
- Dutta, H.; Paul, S.K. Kombucha Drink: Production, Quality, and Safety Aspects. In Production and Management of Beverages; Elsevier: Amsterdam, The Netherlands, 2019; Volume 1, pp. 259–288. [Google Scholar]
- Goh, W.N.; Rosma, A.; Kaur, B.; Fazilah, A.; Karim, A.A.; Bhat, R. Fermentation of Black Tea Broth (Kombucha): I. Effects of Sucrose Concentration and Fermentation Time on the Yield of Microbial Cellulose. Int. Food Res. J. 2012, 19, 109–117. [Google Scholar]
- Abraham, A.G.; DE Antoni, G.L. Characterization of Kefir Grains Grown in Cows’ Milk and in Soya Milk. J. Dairy Res. 1999, 66, 327–333. [Google Scholar] [CrossRef] [PubMed]
- Angulo, L.; Lopez, E.; Lema, C. Microflora Present in Kefir Grains of the Galician Region (North-West of Spain). J. Dairy Res. 1993, 60, 263–267. [Google Scholar] [CrossRef]
- Farnworth, E.R. Kefir—A Complex Probiotic. Food Sci. Technol. Bull. Funct. Foods 2005, 2, 1–17. [Google Scholar] [CrossRef]
- Rosa, D.D.; Dias, M.M.S.; Grześkowiak, Ł.M.; Reis, S.A.; Conceição, L.L.; Maria do Carmo, G.P. Milk Kefir: Nutritional, Microbiological and Health Benefits. Nutr. Res. Rev. 2017, 30, 82–96. [Google Scholar] [CrossRef] [PubMed]
- Farnworth, E.R. Handbook of Fermented Functional Foods, 2nd ed.; CRC Press: Boca Raton, FL, USA, 2008. [Google Scholar]
- Tzavaras, D.; Papadelli, M.; Ntaikou, I. From Milk Kefir to Water Kefir: Assessment of Fermentation Processes, Microbial Changes and Evaluation of the Produced Beverages. Fermentation 2022, 8, 135. [Google Scholar] [CrossRef]
- Pothuraju, R.; Yenuganti, V.R.; Hussain, S.A.; Sharma, M. Fermented Milk in Protection against Inflammatory Mechanisms in Obesity. In Immunity and Inflammation in Health and Disease: Emerging Roles of Nutraceuticals and Functional Foods in Immune Support; Academic Press: Cambridge, MA, USA, 2018; pp. 389–401. [Google Scholar]
- Villarreal-Soto, S.A.; Beaufort, S.; Bouajila, J.; Souchard, J.-P.; Taillandier, P. Understanding Kombucha Tea Fermentation: A Review. J. Food Sci. 2018, 83, 580–588. [Google Scholar] [CrossRef] [PubMed]
- Fiorda, F.A.; Pereira, G.V.D.M.; Thomaz-Soccol, V.; Rakshit, S.K.; Pagnoncelli, M.G.B.; Vandenberghe, L.P.D.S.; Soccol, C.R. Microbiological, Biochemical, and Functional Aspects of Sugary Kefir Fermentation—A Review. Food Microbiol. 2017, 66, 86–95. [Google Scholar] [CrossRef] [PubMed]
- Velicanski, A.; Cvetkovic, D.; Markov, S.; Tumbas, V.; Savatovic, S. Antimicrobial and Antioxidant Activity of Lemon Balm Kombucha. Acta Period. Technol. 2007, 38, 165–172. [Google Scholar] [CrossRef]
- Chakravorty, S.; Bhattacharya, S.; Bhattacharya, D.; Sarkar, S.; Gachhui, R. Kombucha: A Promising Functional Beverage Prepared from Tea. In Non-Alcoholic Beverages; Woodhead Publishing: Cambridge, UK, 2019; Volume 6, pp. 285–327. [Google Scholar] [CrossRef]
- Battikh, H.; Bakhrouf, A.; Ammar, E. Antimicrobial Effect of Kombucha Analogues. LWT 2012, 47, 71–77. [Google Scholar] [CrossRef]
- Vitas, J.S.; Cvetanović, A.D.; Mašković, P.Z.; Švarc-Gajić, J.V.; Malbaša, R.V. Chemical Composition and Biological Activity of Novel Types of Kombucha Beverages with Yarrow. J. Funct. Foods 2018, 44, 95–102. [Google Scholar] [CrossRef]
- Malbaša, R.V.; Milanović, S.D.; Lončar, E.S.; Djurić, M.S.; Carić, M.; Iličić, M.D.; Kolarov, L. Milk-Based Beverages Obtained by Kombucha Application. Food Chem. 2009, 112, 178–184. [Google Scholar] [CrossRef]
- Malbasa, R.; Loncar, E.; Milanovic, S.; Kolarov, L. Use of Milk-Based Kombucha Inoculum for Milk Fermentation. Acta Period. Technol. 2009, 40, 47–52. [Google Scholar] [CrossRef]
- Vukic, V.R.; Hrnjez, D.V.; Kanuric, K.G.; Milanovic, S.D.; Iličic, M.D.; Torbica, A.M.; Tomic, J.M. The Effect of Kombucha Starter Culture on the Gelation Process, Microstructure and Rheological Properties during Milk Fermentation. J. Texture Stud. 2014, 45, 261–273. [Google Scholar] [CrossRef]
- Corona, O.; Randazzo, W.; Miceli, A.; Guarcello, R.; Francesca, N.; Erten, H.; Moschetti, G.; Settanni, L. Characterization of Kefir-like Beverages Produced from Vegetable Juices. LWT 2016, 66, 572–581. [Google Scholar] [CrossRef]
- Mauricio, J. Kefir D’Aqua and Its Probiotic Properties. In Probiotic in Animals; Rigobelo, E., Ed.; InTech: Rijeka, Croatia, 2012. [Google Scholar]
- Rodrigues, K.L.; Araújo, T.H.; Schneedorf, J.M.; Ferreira, C.D.S.; Moraes, G.D.O.I.; Coimbra, R.S.; Rodrigues, M.R. A Novel Beer Fermented by Kefir Enhances Anti-Inflammatory and Anti-Ulcerogenic Activities Found Isolated in Its Constituents. J. Funct. Foods 2016, 21, 58–69. [Google Scholar] [CrossRef]
- Watawana, M.I.; Jayawardena, N.; Waisundara, V.Y. Enhancement of the Functional Properties of Coffee Through Fermentation by “Tea Fungus” (Kombucha). J. Food Process Preserv. 2015, 39, 2596–2603. [Google Scholar] [CrossRef]
- Vimercati, W.C.; Araújo, C.D.S.; Macedo, L.L.; Fonseca, H.C.; Guimarães, J.S.; de Abreu, L.R.; Pinto, S.M. Physicochemical, Rheological, Microbiological and Sensory Properties of Newly Developed Coffee Flavored Kefir. LWT 2020, 123, 109069. [Google Scholar] [CrossRef]
- Puerari, C.; Magalhães, K.T.; Schwan, R.F. New Cocoa Pulp-Based Kefir Beverages: Microbiological, Chemical Composition and Sensory Analysis. Food Res. Int. 2012, 48, 634–640. [Google Scholar] [CrossRef]
- Ayed, L.; Hamdi, M. Manufacture of A Beverage from Cactus Pear Juice Using “Tea Fungus” Fermentation. Ann. Microbiol. 2015, 65, 2293–2299. [Google Scholar] [CrossRef]
- Abuduaibifu, A.; Tamer, C.E. Evaluation of Physicochemical and Bioaccessibility Properties of Goji Berry Kombucha. J. Food Process Preserv. 2019, 43, e14077. [Google Scholar] [CrossRef]
- Watawana, M.I.; Jayawardena, N.; Gunawardhana, C.B.; Waisundara, V.Y. Enhancement of the Antioxidant and Starch Hydrolase Inhibitory Activities of King Coconut Water (Cocos nucifera Var. Aurantiaca) by Fermentation with Kombucha ‘Tea Fungus’. Int. J. Food Sci. Technol. 2016, 51, 490–498. [Google Scholar] [CrossRef]
- Prado, F.C.; Lindner, J.D.D.; Inaba, J.; Thomaz-Soccol, V.; Brar, S.K.; Soccol, C.R. Development and Evaluation of a Fermented Coconut Water Beverage with Potential Health Benefits. J. Funct. Foods 2015, 12, 489–497. [Google Scholar] [CrossRef]
- Yavari, N.; Assadi, M.M.; Larijani, K.; Moghadam, M.B. Response Surface Methodology for Optimization of Glucuronic Acid Production Using Kombucha Layer on Sour Cherry Juice. Aust. J. Basic Appl. Sci. 2010, 4, 3250–3256. [Google Scholar]
- Alsayadi, M.; Al Jawfi, Y.; Belarbi, M.; Sabri, F.Z. Antioxidant of Potency of Water Kefir. J. Microbiol. Biotechnol. Food Sci. 2013, 2021, 2444–2447. [Google Scholar]
- Liamkaew, D.R.; Chattrawanit, J.; Danvirutai, D.P. Kombucha Production by Combinations of Black Tea and Apple Juice. Prog. Appl. Sci. Technol. 2016, 6, 139–146. [Google Scholar]
- Zubaidah, E.; Yurista, S.; Rahmadani, N.R. Characteristic of Physical, Chemical, and Microbiological Kombucha from Various Varieties of Apples. IOP Conf. Ser. Earth Environ. Sci. 2017, 131, 012040. [Google Scholar] [CrossRef]
- Zannini, E.; Lynch, K.M.; Nyhan, L.; Sahin, A.W.; Riordan, P.O.; Luk, D.; Arendt, E.K. Influence of Substrate on the Fermentation Characteristics and Culture-Dependent Microbial Composition of Water Kefir. Fermentation 2022, 9, 28. [Google Scholar] [CrossRef]
- Yavari, N.; Mazaheri-Assadi, M.; Mazhari, Z.H.; Moghadam, M.B.; Larijani, K. Glucuronic Acid Rich Kombucha-fermented Pomegranate Juice. J. Food Res. 2017, 7, 61. [Google Scholar] [CrossRef]
- Kazakos, S.; Mantzourani, I.; Nouska, C.; Alexopoulos, A.; Bezirtzoglou, E.; Bekatorou, A.; Plessas, S.; Varzakas, T. Production of Low-Alcohol Fruit Beverages Through Fermentation of Pomegranate and Orange Juices with Kefir Grains. Curr. Res. Nutr. Food Sci. J. 2016, 4, 19–26. [Google Scholar] [CrossRef]
- Pure, A.E.; Pure, M.E. Antioxidant and Antibacterial Activity of Kombucha Beverages Prepared Using Banana Peel, Common Nettles and Black Tea Infusions. Appl. Food Biotechnol. 2016, 3, 125–130. [Google Scholar] [CrossRef]
- Sharifudin, S.A.; Ho, W.Y.; Yeap, S.K.; Abdullah, R.; Koh, S.P. Fermentation and Characterisation of Potential Kombucha Cultures on Papaya-Based Substrates. LWT 2021, 151, 112060. [Google Scholar] [CrossRef]
- Koh, W.Y.; Utra, U.; Rosma, A.; Effarizah, M.E.; Rosli, W.I.W.; Park, Y.-H. Development of a Novel Fermented Pumpkin-Based Beverage Inoculated with Water Kefir Grains: A Response Surface Methodology Approach. Food Sci. Biotechnol. 2018, 27, 525–535. [Google Scholar] [CrossRef]
- Sirirat, D.; Jelena, P. Bacterial Inhibition and Antioxidant Activity of Kefir Produced from Thai Jasmine Rice Milk. Biotechnology 2010, 9, 332–337. [Google Scholar] [CrossRef]
- Vitas, J.; Malbasa, R.; Grahovac, J.; Loncar, E. The Antioxidant Activity of Kombucha Fermented Milk Products with Stinging Nettle and Winter Savory. Chem. Ind. Chem. Eng. Q. 2013, 19, 129–139. [Google Scholar] [CrossRef]
- Cvetkovic, D.; Markov, S. Preparation of Kombucha from Winter Savory (Satureja montana L.) in the Laboratory Bioreactor. Acta Period. Technol. 2005, 36, 187–196. [Google Scholar] [CrossRef]
- Gamboa-Gómez, C.I.; González-Laredo, R.F.; Gallegos-Infante, J.A.; Pérez, M.D.M.L.; Moreno-Jiménez, M.R.; Flores-Rueda, A.G.; Rocha-Guzmán, N.E. Antioxidant and Angiotensin-Converting Enzyme Inhibitory Activity of Eucalyptus camaldulensis and Litsea glaucescens Infusions Fermented with Kombucha Consortium. Food Technol. Biotechnol. 2016, 54, 367–374. [Google Scholar] [CrossRef] [PubMed]
- Salafzoon, S.; Hosseini, H.M.; Halabian, R. Evaluation of the Antioxidant Impact of Ginger-Based Kombucha on the Murine Breast Cancer Model. J. Complement. Integr. Med. 2018, 15, 9508–9520. [Google Scholar] [CrossRef] [PubMed]
- Belyaev, A.G.; Kaluzhskikh, A.A.; Boev, S.G.; Bashkirev, A.P.; Budnikova, A.S.; Kuleshova, E.S. Research of the Effect of Willow-Herb Products in the Preparation of Kefir on the Composition of Fatty Acids. IOP Conf. Ser. Earth Environ. Sci. 2021, 640, 042006. [Google Scholar] [CrossRef]
- Tu, C.; Tang, S.; Azi, F.; Hu, W.; Dong, M. Use of Kombucha Consortium to Transform Soy Whey into a Novel Functional Beverage. J. Funct. Foods 2018, 52, 81–89. [Google Scholar] [CrossRef]
- Xia, X.; Dai, Y.; Wu, H.; Liu, X.; Wang, Y.; Yin, L.; Wang, Z.; Li, X.; Zhou, J. Kombucha Fermentation Enhances the Health-Promoting Properties of Soymilk Beverage. J. Funct. Foods 2019, 62, 103549. [Google Scholar] [CrossRef]
- Kesenkaş, H. Physicochemical, Microbiological and Sensory Characteristics of Soymilk Kefir. Afr. J. Microbiol. Res. 2011, 5, 3737–3746. [Google Scholar] [CrossRef]
- Belloso-Morales, G.; Hernández-Sánchez, H. Manufacture of a Beverage from Cheese Whey Using a Tea Fungus Fermentation. Rev. Latinoam. De Microbiol. 2003, 45, 5. [Google Scholar]
- Magalhães, K.T.; Dias, D.R.; Pereira, G.; Oliveira, J.; Domingues, L.; Teixeira, J.; Silva, J.B.D.A.E.; Schwan, R.F. Chemical Composition and Sensory Analysis of Cheese Whey-Based Beverages Using Kefir Grains as Starter Culture. Int. J. Food Sci. Technol. 2011, 46, 871–878. [Google Scholar] [CrossRef]
- Kanurić, K.G.; Milanović, S.D.; Ikonić, B.B.; Lončar, E.S.; Iličić, M.D.; Vukić, V.R.; Vukic, D. Kinetics of Lactose Fermentation in Milk with Kombucha Starter. J. Food Drug Anal. 2018, 26, 1229–1234. [Google Scholar] [CrossRef]
- Elkhtab, E.; El-Alfy, M.; Shenana, M.; Mohamed, A.; Yousef, A.E. New Potentially Antihypertensive Peptides Liberated in Milk during Fermentation with Selected Lactic Acid Bacteria and Kombucha Cultures. J. Dairy Sci. 2017, 100, 9508–9520. [Google Scholar] [CrossRef] [PubMed]
- Kruk, M.; Trząskowska, M.; Ścibisz, I.; Pokorski, P. Application of the “SCOBY” and Kombucha Tea for the Production of Fermented Milk Drinks. Microorganisms 2021, 9, 123. [Google Scholar] [CrossRef] [PubMed]
- Güzel-Seydim, Z.B.; Seydim, A.C.; Greene, A.; Bodine, A. Determination of Organic Acids and Volatile Flavor Substances in Kefir during Fermentation. J. Food Compos. Anal. 2000, 13, 35–43. [Google Scholar] [CrossRef]
- Magalhães, K.T.; Pereira, G.V.D.M.; Campos, C.R.; Dragone, G.; Schwan, R.F. Brazilian Kefir: Structure, Microbial Communities and Chemical Composition. Braz. J. Microbiol. 2011, 42, 693–702. [Google Scholar] [CrossRef]
- Iličić, M.; Kanurić, K.; Milanović, S.; Lončar, E.; Djurić, M.; Malbaša, R. Lactose Fermentation by Kombucha—A Process to Obtain New Milk-Based Beverages. Rom. Biotechnol. Lett. 2012, 17, 7013–7021. [Google Scholar]
- Beshkova, D.; Simova, E.; Simov, Z.; Frengova, G.; Spasov, Z. Pure Cultures for Making Kefir. Food Microbiol. 2002, 19, 537–544. [Google Scholar] [CrossRef]
- Fontán, M.C.G.; Martínez, S.; Franco, I.; Carballo, J. Microbiological and Chemical Changes during the Manufacture of Kefir Made from Cows’ Milk, Using a Commercial Starter Culture. Int. Dairy J. 2006, 16, 762–767. [Google Scholar] [CrossRef]
- Kavas, G. Kefirs Manufactured from Camel (Camelus dramedarius) Milk and Cow Milk: Comparison of Some Chemical and Microbial Properties. Ital. J. Food Sci. 2015, 27, 357–365. [Google Scholar]
- Satir, G.; Guzel-Seydim, Z.B. Influence of Kefir Fermentation on the Bioactive Substances of Different Breed Goat Milks. LWT 2015, 63, 852–858. [Google Scholar] [CrossRef]
- Tratnik, L.; Bozanic, R.; Herceg, Z.; Drgalic, I. The Quality of Plain and Supplemented Kefir from Goat’s and Cow’s Milk. Int. J. Dairy Technol. 2006, 59, 40–46. [Google Scholar] [CrossRef]
- Cais-Sokolińska, D.; Wójtowski, J.; Pikul, J. Rheological, Texture and Sensory Properties of Kefir from Mare’s Milk and Its Mixtures with Goat and Sheep Milk. Mljekarstvo 2016, 66, 272–281. [Google Scholar] [CrossRef]
- Cais-Sokolińska, D.; Wójtowski, J.; Pikul, J.; Danków, R.; Majcher, M.; Teichert, J.; Bagnicka, E. Formation of Volatile Compounds in Kefir Made of Goat and Sheep Milk with High Polyunsaturated Fatty Acid Content. J. Dairy Sci. 2015, 98, 6692–6705. [Google Scholar] [CrossRef] [PubMed]
- Kaczyński, K.; Cais-Sokolińska, D.; Rudzińska, M. Cholesterol Oxidation Products in Kefir from Goats’ Milk during Storage. Int. Dairy J. 2018, 85, 35–40. [Google Scholar] [CrossRef]
- Bensmira, M.; Nsabimana, C.; Jiang, B. Effects of Fermentation Conditions and Homogenization Pressure on the Rheological Properties of Kefir. LWT 2010, 43, 1180–1184. [Google Scholar] [CrossRef]
- Wszolek, M.; Tamime, A.; Muir, D.; Barclay, M. Properties of Kefir Made in Scotland and Poland Using Bovine, Caprine and Ovine Milk with Different Starter Cultures. LWT 2001, 34, 251–261. [Google Scholar] [CrossRef]
- Amarasinghe, H.; Weerakkody, N.; Waisundara, V.Y. Evaluation of Physicochemical Properties and Antioxidant Activities of Kombucha “Tea Fungus” during Extended Periods of Fermentation. Food Sci. Nutr. 2018, 6, 659–665. [Google Scholar] [CrossRef]
- Barbosa, C.D.; Uetanabaro, A.P.T.; Santos, W.C.R.; Caetano, R.G.; Albano, H.; Kato, R.; Cosenza, G.P.; Azeredo, A.; Góes-Neto, A.; Rosa, C.A.; et al. Microbial–Physicochemical Integrated Analysis of Kombucha Fermentation. LWT 2021, 148, 111788. [Google Scholar] [CrossRef]
- Bauer-Petrovska, B.; Petrushevska-Tozi, L. Mineral and Water Soluble Vitamin Content in the Kombucha Drink. Int. J. Food Sci. Technol. 2000, 35, 201–205. [Google Scholar] [CrossRef]
- Chakravorty, S.; Bhattacharya, S.; Chatzinotas, A.; Chakraborty, W.; Bhattacharya, D.; Gachhui, R. Kombucha Tea Fermentation: Microbial and Biochemical Dynamics. Int. J. Food Microbiol. 2016, 220, 63–72. [Google Scholar] [CrossRef]
- Chen, C.; Liu, B. Changes in Major Components of Tea Fungus Metabolites during Prolonged Fermentation. J. Appl. Microbiol. 2000, 89, 834–839. [Google Scholar] [CrossRef]
- Gaggìa, F.; Baffoni, L.; Galiano, M.; Nielsen, D.S.; Jakobsen, R.R.; Castro-Mejía, J.L.; Bosi, S.; Truzzi, F.; Musumeci, F.; Dinelli, G.; et al. Kombucha Beverage from Green, Black and Rooibos Teas: A Comparative Study Looking at Microbiology, Chemistry and Antioxidant Activity. Nutrients 2018, 11, 1. [Google Scholar] [CrossRef]
- Ivanišová, E.; Meňhartová, K.; Terentjeva, M.; Harangozo, L.; Kántor, A.; Kačániová, M. The Evaluation of Chemical, Antioxidant, Antimicrobial and Sensory Properties of Kombucha Tea Beverage. J. Food Sci. Technol. 2019, 57, 1840–1846. [Google Scholar] [CrossRef] [PubMed]
- Jafari, R.; Naghavi, N.S.; Khosravi-Darani, K.; Doudi, M.; Shahanipour, K. Kombucha Microbial Starter with Enhanced Production of Antioxidant Compounds and Invertase. Biocatal. Agric. Biotechnol. 2020, 29, 101789. [Google Scholar] [CrossRef]
- Jayabalan, R.; Marimuthu, S.; Swaminathan, K. Changes in Content of Organic Acids and Tea Polyphenols during Kombucha Tea Fermentation. Food Chem. 2007, 102, 392–398. [Google Scholar] [CrossRef]
- Kaewkod, T.; Bovonsombut, S.; Tragoolpua, Y. Efficacy of Kombucha Obtained from Green, Oolong, and Black Teas on Inhibition of Pathogenic Bacteria, Antioxidation, and Toxicity on Colorectal Cancer Cell Line. Microorganisms 2019, 7, 700. [Google Scholar] [CrossRef]
- Muhialdin, B.J.; Osman, F.A.; Muhamad, R.; Wan Sapawi, C.W.N.S.C.; Anzian, A.; Voon, W.W.Y.; Hussin, A.S.M. Effects of Sugar Sources and Fermentation Time on the Properties of Tea Fungus (Kombucha) Beverage. Int. Food Res. J. 2019, 26, 481–487. [Google Scholar]
- Neffe-Skocińska, K.; Sionek, B.; Ścibisz, I.; Kolozyn-Krajewska, D. Acid contents and the Effect of Fermentation Condition of Kombucha Tea Beverages on Physicochemical, Microbiological and Sensory Properties. CyTA J. Food 2017, 15, 601–607. [Google Scholar] [CrossRef]
- Sharma, C.; Bhardwaj, N.K. Biotransformation of Fermented Black Tea into Bacterial Nanocellulose via Symbiotic Interplay of Microorganisms. Int. J. Biol. Macromol. 2019, 132, 166–177. [Google Scholar] [CrossRef]
- Watawana, M.I.; Jayawardena, N.; Waisundara, V.Y. Value-Added Tea (Camellia sinesis) as a Functional Food Using the Kombucha ‘Tea Fungus. Warasan Khana Witthayasat Maha Witthayalai Chiang Mai 2018, 45, 136–146. [Google Scholar]
- Fabricio, M.F.; Mann, M.B.; Kothe, C.I.; Frazzon, J.; Tischer, B.; Flôres, S.H.; Ayub, M.A.Z. Effect of Freeze-Dried Kombucha Culture on Microbial Composition and Assessment of Metabolic Dynamics during Fermentation. Food Microbiol. 2021, 101, 103889. [Google Scholar] [CrossRef] [PubMed]
- Jakubczyk, K.; Kałduńska, J.; Kochman, J.; Janda, K. Chemical Profile and Antioxidant Activity of the Kombucha Beverage Derived from White, Green, Black and Red Tea. Antioxidants 2020, 9, 447. [Google Scholar] [CrossRef] [PubMed]
- Lee, S.-P.; Kim, C.-S. Characterization of Kombucha Beverages Fermented with Various Teas and Tea Fungus. J. Food Sci. Nutr. 2000, 5, 165–169. [Google Scholar]
- Treviño-Garza, M.Z.; Guerrero-Medina, A.S.; González-Sánchez, R.A.; García-Gómez, C.; Guzmán-Velasco, A.; Báez-González, J.G.; Márquez-Reyes, J.M. Production of Microbial Cellulose Films from Green Tea (Camellia sinensis) Kombucha with Various Carbon Sources. Coatings 2020, 10, 1132. [Google Scholar] [CrossRef]
- Loncar, E.; Malbasa, R.; Kolarov, L. Kombucha Fermentation on Raw Extracts of Different Cultivars of Jerusalem Artichoke. Acta Period. Technol. 2007, 38, 37–44. [Google Scholar] [CrossRef]
- Nugraha, T.; Susilowati, A.; Aspiyanto; Lotulung, P.D.; Maryati, Y. Characterization of Biomasses, Concentrates, and Permeates of Dried Powder of Kombucha Fermentation of Spinach (Amaranthus sp.) and Broccoli (Brassica oleracea) with Membrane Microfiltration and Freeze Drying Techniques for Natural Sources of Folic Acid. In Proceedings of the 3rd International Symposium on Applied Chemistry 2017, Jakarta, Indonesia, 23–24 October 2017. [Google Scholar] [CrossRef]
- Uțoiu, E.; Matei, F.; Toma, A.; Diguță, C.F.; Ștefan, L.M.; Mănoiu, S.; Vrăjmașu, V.V.; Moraru, I.; Oancea, A.; Israel-Roming, F.; et al. Bee Collected Pollen with Enhanced Health Benefits, Produced by Fermentation with a Kombucha Consortium. Nutrients 2018, 10, 1365. [Google Scholar] [CrossRef] [PubMed]
- Doğan, M. Rheological Behaviour and Physicochemical Properties of Kefir with Honey. J. Consum. Prot. Food Saf. 2010, 6, 327–332. [Google Scholar] [CrossRef]
- Emiljanowicz, K.E.; Malinowska-Pańczyk, E. Kombucha from Alternative Raw Materials—The Review. Crit. Rev. Food Sci. Nutr. 2020, 60, 3185–3194. [Google Scholar] [CrossRef]
- Jayabalan, R.; Malbaša, R.V.; Lončar, E.S.; Vitas, J.S.; Sathishkumar, M. A Review on Kombucha Tea-Microbiology, Composition, Fermentation, Beneficial Effects, Toxicity, and Tea Fungus. Compr. Rev. Food Sci. Food Saf. 2014, 13, 538–550. [Google Scholar] [CrossRef]
- Teoh, A.L.; Heard, G.; Cox, J. Yeast Ecology of Kombucha Fermentation. Int. J. Food Microbiol. 2004, 95, 119–126. [Google Scholar] [CrossRef]
- May, A.; Narayanan, S.; Alcock, J.; Varsani, A.; Maley, C.; Aktipis, A. Kombucha: A Novel Model System for Cooperation and Conflict in a Complex Multi-Species Microbial Ecosystem. Peer J. 2019, 7, e7565. [Google Scholar] [CrossRef] [PubMed]
- Villarreal-Soto, S.A.; Bouajila, J.; Pace, M.; Leech, J.; Cotter, P.D.; Souchard, J.-P.; Taillandier, P.; Beaufort, S. Metabolome-Microbiome Signatures in the Fermented Beverage, Kombucha. Int. J. Food Microbiol. 2020, 333, 108778. [Google Scholar] [CrossRef] [PubMed]
- Coton, M.; Pawtowski, A.; Taminiau, B.; Burgaud, G.; Deniel, F.; Coulloumme-Labarthe, L.; Fall, A.; Daube, G.; Coton, E. Unraveling Microbial Ecology of Industrial-Scale Kombucha Fermentations by Metabarcoding and Culture-Based Methods. FEMS Microbiol. Ecol. 2017, 93, fix048. [Google Scholar] [CrossRef] [PubMed]
- Gao, X.; Li, B. Chemical and Microbiological Characteristics of Kefir Grains and Their Fermented Dairy Products: A Review. Cogent Food Agric. 2016, 2, 1272152. [Google Scholar] [CrossRef]
- Rattray, F.P.; O’Connell, M.J. Fermented Milks|Kefir. In Encyclopedia of Dairy Sciences; Academic Press: San Diego, CA, USA, 2011; pp. 518–524. [Google Scholar]
- Sharma, H.; Ozogul, F.; Bartkiene, E.; Rocha, J.M. Impact of Lactic Acid Bacteria and Their Metabolites on the Techno-Functional Properties and Health Benefits of Fermented Dairy Products. Crit. Rev. Food Sci. Nutr. 2021, 1–23. [Google Scholar] [CrossRef] [PubMed]
- Harutoshi, T. Exopolysaccharides of Lactic Acid Bacteria for Food and Colon Health Applications. In Lactic Acid Bacteria-R & D for Food, Health and Livestock Purposes; IntechOpen: London, UK, 2013. [Google Scholar]
- Alves, E.; Ntungwe, E.N.; Gregório, J.; Rodrigues, L.M.; Pereira-Leite, C.; Caleja, C.; Pereira, E.; Barros, L.; Aguilar-Vilas, M.V.; Rosado, C.; et al. Characterization of Kefir Produced in Household Conditions: Physicochemical and Nutritional Profile, and Storage Stability. Foods 2021, 10, 1057. [Google Scholar] [CrossRef]
- Leite, A.; Leite, D.; Del Aguila, E.; Alvares, T.; Peixoto, R.; Miguel, M.; Silva, J.; Paschoalin, V. Microbiological and Chemical Characteristics of Brazilian Kefir during Fermentation and Storage Processes. J. Dairy Sci. 2013, 96, 4149–4159. [Google Scholar] [CrossRef]
- Farag, M.A.; Jomaa, S.A.; El-Wahed, A.A.; El-Seedi, H.R. The Many Faces of Kefir Fermented Dairy Products: Quality Characteristics, Flavour Chemistry, Nutritional Value, Health Benefits, and Safety. Nutrients 2020, 12, 346. [Google Scholar] [CrossRef]
- Tozakidou, N. Comparative Nutritional Study of Several “Fermented” Drinks: Fassbrause, Kombucha, Cider, Water Kefir. Fabrication, Analysis (Rate of Alcohol, Proteins, Minerals), Sensory Analysis (Descriptors), Microbiology Analysis. Bachelor’s Thesis, Technological University Institute of Lille “IUT 1”, Villeneuve-d’Ascq, France, 2017. [Google Scholar]
- Diosma, G.; Romanin, D.E.; Rey-Burusco, M.F.; Londero, A.; Garrote, G.L. Yeasts from Kefir Grains: Isolation, Identification, and Probiotic Characterization. World J. Microbiol. Biotechnol. 2013, 30, 43–53. [Google Scholar] [CrossRef]
- Chen, Z.; Shi, J.; Yang, X.; Nan, B.; Liu, Y.; Wang, Z. Chemical and Physical Characteristics and Antioxidant Activities of the Exopolysaccharide Produced by Tibetan Kefir Grains during Milk Fermentation. Int. Dairy J. 2015, 43, 15–21. [Google Scholar] [CrossRef]
- Marsh, A.J.; O’Sullivan, O.; Hill, C.; Ross, R.P.; Cotter, P.D. Sequence-Based Analysis of the Bacterial and Fungal Compositions of Multiple Kombucha (Tea Fungus) Samples. Food Microbiol. 2013, 38, 171–178. [Google Scholar] [CrossRef] [PubMed]
- Wang, S.; Zhang, L.; Qi, L.; Liang, H.; Lin, X.; Li, S.; Yu, C.; Ji, C. Effect of Synthetic Microbial Community on Nutraceutical and Sensory Qualities of Kombucha. Int. J. Food Sci. Technol. 2020, 55, 3327–3333. [Google Scholar] [CrossRef]
- Mayser, P.; Fromme, S.; Leitzmann, G.; Gründer, K. The Yeast Spectrum of the ‘Tea Fungus Kombucha’. Mycoses 1995, 38, 289–295. [Google Scholar] [CrossRef]
- Hopfe, S.; Flemming, K.; Lehmann, F.; Möckel, R.; Kutschke, S.; Pollmann, K. Leaching of Rare Earth Elements from Fluorescent Powder Using the Tea Fungus Kombucha. Waste Manag. 2017, 62, 211–221. [Google Scholar] [CrossRef] [PubMed]
- Jayabalan, R.; Malini, K.; Sathishkumar, M.; Swaminathan, K.; Yun, S.-E. Biochemical Characteristics of Tea Fungus Produced during Kombucha Fermentation. Food Sci. Biotechnol. 2010, 19, 843–847. [Google Scholar] [CrossRef]
- Angela, C.; Young, J.; Kordayanti, S.; Devanthi, P.V.P. Isolation and Screening of Microbial Isolates from Kombucha Culture for Bacterial Cellulose Production in Sugarcane Molasses Medium. In Proceedings of the 2019 International Conference on Biotechnology and Life Sciences (IC-BIOLIS), Jakarta, Indonesia, 8–9 October 2019. [Google Scholar]
- Ramadani, A.S.; Abulreesh, H.H. Isolation and Identification of Yeast Flora in Local Kombucha Sample: AL NABTAH. J. App. Sci. 2010, 2, 42–51. [Google Scholar]
- Watawana, M.I.; Jayawardena, N.; Ranasinghe, S.J.; Waisundara, V.Y. Evaluation of the Effect of Different Sweetening Agents on the Polyphenol Contents and Antioxidant and Starch Hydrolase Inhibitory Properties of Kombucha. J. Food Process Preserv. 2016, 41, e12752. [Google Scholar] [CrossRef]
- Huang, X.; Xin, Y.; Lu, T. A Systematic, Complexity-Reduction Approach to Dissect the Kombucha Tea Microbiome. Elife 2022, 11, e76401. [Google Scholar] [CrossRef]
- Garrote, G.L.; Abraham, A.G.; DE Antoni, G.L. Chemical and Microbiological Characterisation of Kefir Grains. J. Dairy Res. 2001, 68, 639–652. [Google Scholar] [CrossRef]
- Dobson, A.; O’Sullivan, O.; Cotter, P.D.; Ross, R.; Hill, C. High-Throughput Sequence-Based Analysis of the Bacterial Composition of Kefir and an Associated Kefir Grain. FEMS Microbiol. Lett. 2011, 320, 56–62. [Google Scholar] [CrossRef]
- Wyder, M.T.; Spillmann, H.; Puhan, Z. Investigation of the Yeast Flora in Dairy Products: A Case Study of Kefyr. Food Technol. Biotechnol. 1997, 35, 299–304. [Google Scholar]
- Wyder, M.-T.; Meile, L.; Teuber, M. Description of Saccharomyces turicensis sp. nov., a New Species from Kefyr. Syst. Appl. Microbiol. 1999, 22, 420–425. [Google Scholar] [CrossRef]
- Kumar, M.R.; Yeap, S.K.; Mohamad, N.E.; Abdullah, J.O.; Masarudin, M.J.; Khalid, M.; Leow, A.T.C.; Alitheen, N.B. Metagenomic and Phytochemical Analyses of Kefir Water and Its Subchronic Toxicity Study in BALB/c Mice. BMC Complement. Med. Ther. 2021, 21, 83. [Google Scholar] [CrossRef] [PubMed]
- Tsao, R. Chemistry and Biochemistry of Dietary Polyphenols. Nutrients 2010, 2, 1231–1246. [Google Scholar] [CrossRef] [PubMed]
- Suetsuna, K.; Ukeda, H.; Ochi, H. Isolation and Characterization of Free Radical Scavenging Activities Peptides Derived from Casein. J. Nutr. Biochem. 2000, 11, 128–131. [Google Scholar] [CrossRef]
- Lobo, R.O.; Dias, F.O.; Shenoy, C.K. Kombucha for Healthy Living: Evaluation of Antioxidant Potential and Bioactive Compounds. Int. Food Res. J. 2017, 24, 541–546. [Google Scholar]
- Srihari, T.; Satyanarayana, U. Changes in Free Radical Scavenging Activity of Kombucha during Fermentation. J. Pharm. Sci. Res. 2012, 4, 1978–1981. [Google Scholar]
- Manach, C.; Scalbert, A.; Morand, C.; Rémésy, C.; Jiménez, L. Polyphenols: Food Sources and Bioavailability. Am. J. Clin. Nutr. 2004, 79, 727–747. [Google Scholar] [CrossRef]
- Yokogoshi, H.; Kato, Y.; Sagesaka, Y.M.; Takihara-Matsuura, T.; Kakuda, T.; Takeuchi, N. Reduction Effect of Theanine on Blood Pressure and Brain 5-Hydroxyindoles in Spontaneously Hypertensive Rats. Biosci. Biotechnol. Biochem. 1995, 59, 615–618. [Google Scholar] [CrossRef]
- Xu, S.; Wang, Y.; Wang, J.; Geng, W. Kombucha Reduces Hyperglycemia in Type 2 Diabetes of Mice by Regulating Gut Microbiota and Its Metabolites. Foods 2022, 11, 754. [Google Scholar] [CrossRef]
- Rodrigues, K.L.; Caputo, L.R.G.; Carvalho, J.C.T.; Evangelista, J.; Schneedorf, J.M. Antimicrobial and Healing Activity of Kefir and Kefiran Extract. Int. J. Antimicrob. Agents 2005, 25, 404–408. [Google Scholar] [CrossRef] [PubMed]
- Su, H.; Xie, L.; Xu, Y.; Ke, H.; Bao, T.; Li, Y.; Chen, W. Pelargonidin-3-O-glucoside Derived from Wild Raspberry Exerts Antihyperglycemic Effect by Inducing Autophagy and Modulating Gut Microbiota. J. Agric. Food Chem. 2020, 68, 13025–13037. [Google Scholar] [CrossRef] [PubMed]
- Ibacache-Quiroga, C.; González-Pizarro, K.; Charifeh, M.; Canales, C.; Díaz-Viciedo, R.; Schmachtenberg, O.; Dinamarca, M.A. Metagenomic and Functional Characterization of Two Chilean Kefir Beverages Reveals a Dairy Beverage Containing Active Enzymes, Short-Chain Fatty Acids, Microbial β-Amyloids, and Bio-Film Inhibitors. Foods 2022, 11, 900. [Google Scholar] [CrossRef] [PubMed]
Leave a Comment